Nestled into a hillside of ponderosa pines with views of Humphrey’s Peak, the High-Performance training facility attracts top student-athletes to Northern Arizona University. DLR Group delivered a 65,000 square-foot, three-story, state-of-the-art High-Performance Center with a 10,000 square-foot weight room, sports medicine spaces, academic center, practice basketball courts, team meeting rooms, locker rooms, and a hall of fame. The facility also has an iconic team auditorium on the third level, with unobstructed views of the 12,600-foot Humphrey’s Peak and the San Francisco Range (Figure 1). The unique spaces and environment provided structural challenges and opportunities for creative design.
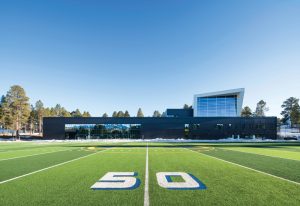
Structural Design Creativity
Incorporating high-performance features and honoring the environment demanded creativity in the structural design of the athletic center. For example, to achieve unobstructed views of Humphrey’s Peak, the orientation of the third-level auditorium and hall of fame, referred to as the Jewel Box, was strategically rotated 15 degrees (Figure 2). The rotation, causing geometric and cost challenges to the structure and design, required the design team to explore multiple framing options.
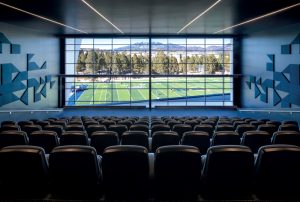
In its initial concept, the Jewel Box was framed as a truss, independent from the rest of the structure. The truss design, however, became expensive and cumbersome as it was challenging to coordinate the truss webs with the floor plan. The budget constraints associated with the initial truss design led to the next solution – a pre-engineered metal building (PEMB). However, the Jewel Box’s extensive cantilevers and sloped geometry made the PEMB inefficient.
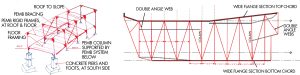
The Jewel Box was ultimately framed with conventional steel (Figure 3), and the team achieved cost savings in the project by having the gym and the weight room constructed as a PEMB. This hybrid framing system worked creatively to deliver on the program requirements and the unique, high-performance spaces within the athletic center. An innovative hybrid of PEMB and conventional framing provided a world-class design in a manner that delivered on budget but did not compromise the overall architectural design and high-performance needs (Figure 4). In addition, the creative use of the exterior paneling and curtain walls ensured building continuity, elevated these otherwise conventional systems, and provided a cohesive look and design.
The Jewel Box’s six columns punctured programming spaces on various levels below, requiring extensive coordination. One of the most critical column locations occurred in the hydrotherapy room. The hydrotherapy room houses pools on the first level, with a basement level to store all the pool equipment. The basement for the hydrotherapy room was structurally framed with 10.5-foot-tall retaining walls and 18-foot-wide footings. The Jewel Box columns extended from the third floor down to the first floor, where they were supported on 24-inch-square concrete piers that extended down to the same footing elevation as the retaining walls. The Jewel Box’s steel columns had to be strategically positioned to avoid the retaining walls.
Complexities and Unique Problems
The collaboration of all structural systems, delegated systems, architectural and mechanical needs, program requirements, and exterior systems created complexities and unique problems in all design phases. As the structural engineer of record, DLR Group managed connections, deflection requirements, and loading between many parties while providing a cohesive design.
The success was largely attributed to an amplified focus on performance criteria. An example of this can be seen in the connections of the wall paneling system. The structural team coordinated the expected 0.07-inch deflection of the 12-foot cantilevered retaining walls with the connection requirements of the 2½-inch insulated wall panels and the expected ¾-inch drift of the steel building superstructure.
The steel columns were placed 1 inch from the retaining wall, and custom wind girt connections were designed for the metal wall panels. HSS wind girts were placed at 8-foot vertical increments – coinciding with the height of the insulated metal wall panels. The custom connection from the HSS wind girts to the panels resulted in an easy installation anchor point for the contractor. The connection of these different systems, each designed by different parties under the guidance of the structural team, created an urgent need for constant communication to anticipate and resolve any constructability issues and ensure the performance criteria were met (Figure 5).
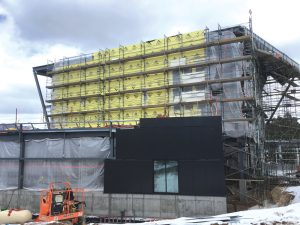
Material and Labor Efficiencies
The pedestrian bridge entry to the third level celebrates the landscape while giving visitors a dramatic approach through the pines as they enter. It also promotes ingenuity of design for efficient use of materials and labor. Located on a hillside, the Student Athlete High-Performance Center has its north side adjacent to the existing football practice field and its south side against the hillside. The university required access to the third-level Jewel Box from the south side of the building.
The exiting grades at the south side of the building did not coincide with the required finish floor elevation, so the team first explored backfilling the soil. However, raising the grade on the south side had other detrimental consequences, including 30-foot-tall retaining walls, large amounts of fill import, loss of daylight into the second floor, and the removal of mature ponderosa pines. Therefore, the structural team worked with the topography instead of against it and provided a precast concrete bridge to alleviate and solve all these problems.
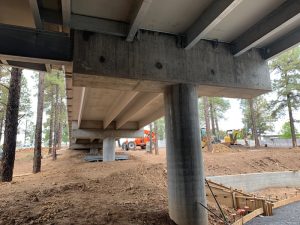
Using the 138-foot-long pedestrian bridge as a design solution provided massive cost savings and schedule benefits (Figure 6). The retaining wall height was reduced to 15 feet, leaving the existing grade vastly undisturbed, gaining daylight at the second floor, and keeping the mature ponderosas in place. Furthermore, a precast patio, benches, and donor monument signs were added to the 20-foot-wide pedestrian bridge, promoting a mall concept where athletes, faculty, and staff could gather before an event. Originally a challenge, access to the third floor has now become a design feature that allows visitors to walk through the trees as they approach the building (Figure 7).
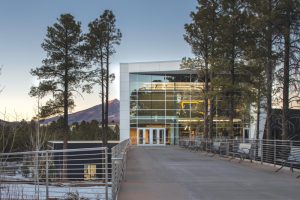
Constructability Challenges
The interaction of the topography and bridge foundations required creative design and quick solutions even after construction had begun, as the bridge foundations interfered with several of the existing ponderosa pines integral to the building’s entrance. The bridge’s 36-inch round columns are designed to be supported by 9-foot by 9-foot by 2-foot concrete spread footings. During construction, it was discovered that the designed footing intruded on a few existing ponderosa pines. The contractor immediately requested a redesign of the footing to allow the existing pines to remain since those pines were strategic to the design concept of entering the building through the pines. The structural team quickly reviewed the foundations for new locations with eccentric loading and provided new designs during construction.
To combat Flagstaff’s cold and snowy winters, the contractor requested an early foundation package to pour before the frost set in, creating design and constructability challenges. The structural team designed foundations without completing the design of the structure, while external designers completed the steel and PEMB design. Thus, loading and layouts could not be guaranteed, which heightened the design challenges.
Addressing these concerns, the structural team worked with the architects to lock down grid locations and loading early. The team increased all initial reactions by 20% and limited foundations to a 50% design/capacity ratio. The resulting combination of these two strategies led to minimal changes to the foundations during construction once the final reactions were computed and backchecked against the existing footing design. Any changes that did occur during construction were more efficiently managed and overcome due to this foresight.
Conclusion
Northern Arizona University’s Student Athlete High-Performance Center showcases structural engineering excellence. Many things make this building unique, such as its location on a hillside, its Jewel Box rotated to maximize views, and the pedestrian entrance to the third level. As a result of these challenges, the engineering team had to perform at higher altitudes to design solutions on budget, use materials efficiently, and overcome construction challenges.■
The author would like to thank Mark Forman, DLR Group, who was the Structural Engineer of Record of the project and Stanley L. Axthem, DLR Group, who was the Architect of Record.
Project Team
Owner: Northern Arizona University
Structural Engineer of Record: DLR Group
Architect of Record: DLR Group
Contractor: Core Construction
Structural Software used: RAM Structural Systems, RAM Connection, RAM Elements, Enercalc, RetainPro