Seismic Excellence Through Base Isolation
The New Stanford Hospital (NSH), designed by Rafael Viñoly Architects, has replaced an existing aging facility with a modern base-isolated structure at the Stanford University Campus. The new seven-story state-of-the-art Level 1 trauma center includes 368 patient rooms, 20 operating suites, imaging and radiology suites, and an emergency department. Following the latest healthcare trend, the hospital was designed to be patient-centric, with patient rooms located around the perimeter of four towers. All rooms are private, with large floor-to-ceiling windows overlooking the surrounding campus and foothills.
A three-story common podium joins the towers and provides a 4-acre green roof terrace accessible for patients and visitors to enjoy. A large column-free atrium with a 120-foot-diameter glazed dome roof is the centerpiece of the public spaces at the podium. A sky bridge and below-grade tunnel connect the new hospital to the adjacent existing hospital, and a helipad is located atop one of the patient towers. The project has several unique aspects, including a new base isolation system, a daring dome structure, extensively cantilevered floor plates, and a novel base-isolated pedestrian sky bridge.
Structural System and Resiliency
The structural system consists of a base-isolated steel bi-directional moment frame utilizing steel box columns and Welded Unreinforced Flange (WUF-W) moment frame connections with concrete slab on metal deck diaphragms. The system was designed with seismic performance and resiliency in mind. The incorporation of base isolation substantially reduces the amount of earthquake energy imparted on the building during an earthquake. Additionally, the moment frame system was designed to be essentially elastic (R = 1) in a Maximum Credible Event (MCE). This provided the dual benefits of eliminating expected damage during a major earthquake and allowing more architectural freedom where prescriptive code requirements could be relaxed.
Additionally, the resiliency-based design enhanced protection for critical non-structural elements like building cladding, medical equipment, and Mechanical, Electrical, and Plumbing (MEP) systems needed for a functional hospital following a major seismic event. The California Building Code (CBC) requires hospitals to be designed to a higher standard than a typical building, and the New Stanford Hospital was designed to an even higher standard with the goals of protecting the Client’s investment and ensuring that the hospital was fully functional to the community after a major earthquake.
A nonlinear response history analysis was utilized to design the structure at the Design Earthquake (DE) and MCE levels. A building model was created in ETABS to evaluate the superstructure, and a separate detailed finite element sub-model was created in SAP2000 to evaluate the base isolator connection to the superstructure. The detailed sub-model proved to be more complicated as it had to consider multiple scenarios, including the P-Delta forces resulting from earthquake displacements in the base isolator and a post-earthquake scenario where the building would need to be jacked up should an isolator bearing require replacement.
Base Isolation
The project was one of the first in the world to use Triple Friction Pendulum isolation bearings, and extensive work was done to validate how to model and test these new bearings. The project also relied on recent research performed on similar bearings tested in a full-scale building at the E-Defense shake table near Kobe, Japan, to provide valuable modeling input and verify the real-world performance.
The bearing manufacturer, Earthquake Protection Systems, performed real-time bearing prototype testing to determine the bearing properties used in the design. Upper and lower bound isolator properties were developed to be used in the analysis to capture potential performance variability of the isolators under different loading conditions. Performing prototype testing early allowed the actual isolator properties to be used during design rather than using an assumed range which would have been more conservative and added cost. All bearings installed in the building also went through production testing to ensure their performance was within the specified limits and the production results matched the prototype results.
This testing and analysis program produced a base isolation system that allows the building to move up to 37 inches horizontally in any direction during an earthquake. A void space known as a base isolation moat completely surrounds the basement allowing the building to move unimpeded horizontally. Additionally, because of the geometry of the Triple Friction Pendulum bearings, the building will rise up to 3 inches vertically as it travels horizontally.
A detailed system of moat covers was provided to bridge the moat around the building to interface with the adjacent sitescape. The moat covers were designed to remain operational over the range of base-isolated structure movements and support a variety of site finishes and pedestrian and vehicular traffic loads – a challenging and creative aspect of the project. The moat covers articulate to keep the moats covered before, during, and after an earthquake while still allowing the abovementioned movements. The covers also needed to match the architecture and preferably be invisible and watertight. The typical moat cover consists of a pop-up assembly provided by Construction Specialties that will articulate and pop up during an earthquake and return to its original at-rest position when shaking stops. The typical moat cover consists of a hinged metal pan filled with concrete and topped with other finishes to blend in with the surrounding site.
The extensive set of utilities required to serve a modern hospital connect to the building within the moat and use a system of flexible couplings and ball joints to accommodate the large differential movements between the base-isolated structure and the adjoining fixed-site during an earthquake, without interruption or damage. One of the project’s successes was the early involvement of Mechanical, Electrical, and Plumbing subcontractors and their active participation in detailing and coordinating the large clearances required around MEP utilities crossing the isolation plane.
Cantilevered Floors
An immediately noticeable aspect of the hospital architecture is the large number of cantilevers throughout the building (Figure 1). Over one-third of the overall building floor is cantilevered to achieve this architectural vision. In addition, the perimeter bay of all four patient towers and most of the second-floor perimeter bays are cantilevered 29 feet beyond the last column line. Each cantilever condition was different, requiring a unique structural solution to achieve the cantilever.
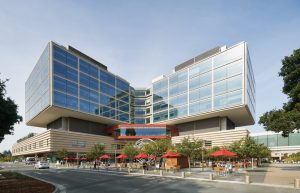
At the patient towers, where four stories of the perimeter framing bays are cantilevered 29 feet beyond the last column line, vertical Vierendeel frames are used at each gridline for support. This Vierendeel system, combined with the bi-axial moment frames at the tower cores, resulted in essentially every beam-column connection of the towers being moment-connected. This interconnection required the entire patient tower to be considered in an ETABS lateral analysis model when designing for gravity and seismic loading.
A mix of diagonal tension hangers at the corners and custom plate girders at the typical bays were used at the second floor to achieve the similarly long cantilevers. In particular, the double cantilevered corner conditions proved to be complicated and challenging to design. They required a second system of perpendicular cantilevers at the corner supported from the typical floor cantilever systems.
These extensive building cantilevers were temporarily shored as part of the initial steel erection. The release of shoring and overall construction sequencing, including the timing and build-out of finishes, interior construction, and exterior cladding systems, was carefully coordinated with the contractor to protect drift and movement-sensitive non-structural systems.
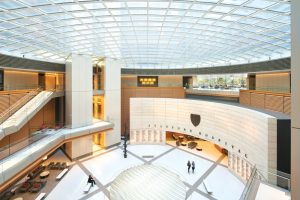
Atrium Dome Structure
The main entrance to the NSH hospital opens into a striking 120-foot-diameter column-free atrium filled with natural light (Figure 2). This is a centerpiece of the hospital and is covered by a glass dome skylight to allow light to reach the occupants below. The structure of the dome is composed of a grid of 5-inch-diameter curved steel ribs surrounded by a circular tension ring at the perimeter of the dome. The ribs are located on an orthogonal grid in plan and are curved vertically to follow the dome’s profile. At the center of the dome, the ribs rise a maximum of 12 feet, creating a gravity-defying thin lens assembly. A series of articulated and repeating glazing panels sit on top of the structure, forming the skylight. The analysis of the dome used the program SAP2000 (Figure 3) and considered construction loading, construction sequencing, and penetrations for elevator towers and tower crane leave-out panels. Designing around these discontinuities to the symmetry and regular geometry of the dome proved to be one of the significant challenges of the dome design.
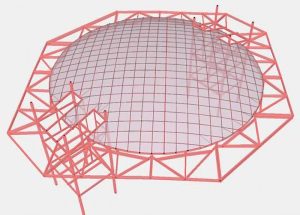
The steel fabricator chose to sub-contract the dome steel fabrication to a specialized fabricator with experience in curved HSS fabrication and construction in the roller coaster and amusement theme park industry to meet the tighter construction tolerances required for the dome construction. The dome was test fit and coordinated with the glazing sub-contractor in the steel shop prior to the eventual successful erection and installation on site.
Pedestrian Skybridge
An innovative long-span pedestrian sky bridge connects the new hospital to the existing hospital at the second floor (Figure 4). A first-of-its-kind structural system was developed to allow the sky bridge to be laterally supported by the new isolated hospital at one end and by the existing fixed-base hospital at the other end while accommodating up to 4 feet of differential seismic movement between the two structures without a large seismic joint. A traditional approach would have used a very large (4 feet in any direction) seismic joint between the isolated and non-isolated structures, which would have been challenging to implement architecturally and taken up valuable space. Instead, the bridge is base-isolated and uses an articulated three-pin dogleg configuration in plan to convert the large seismic movements of the hospital into small rotations at each of the three joints.
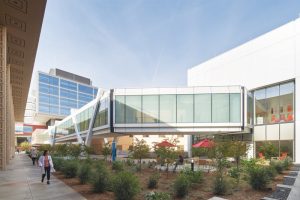
This approach effectively transformed the irregular shape of the bridge into a structural benefit to the project. The resulting joint size is only 3 inches at each of the three articulating joints, yet the bridge can accommodate the full displacement capacity of the hospital isolation bearings. In addition, the base of the bridge is supported by custom and first-of-this-size Tension-Capable cross linear slider isolation bearings, also by Earthquake Protection Systems. These bearings simultaneously resist overturning in the bridge while allowing the bridge to move with the hospital.
Vibration Analysis
The project was required to meet stringent vibration criteria for occupant comfort and imaging performance. Extensive vibration analysis was performed to evaluate nearly all floor areas, including the significant cantilevers described above, and ensure the floor systems met the criteria. Typical framing bays were evaluated using the program FloorVibe, but irregular and cantilever conditions required a more advanced approach. In those cases, a SAP2000 model of the structure was developed, and custom vertical time history forcing functions were applied to the model to emulate the footfall of one or more walkers on the floor. Careful post-processing of the velocity and acceleration data allowed comparison to the project vibration criteria.
The MRI suites posed a unique challenge as they are typically located on a slab on grade to take advantage of the inherent vibration performance of an at-grade condition. However, this was not an option due to the use of base isolation and the presence of the crawlspace below the isolation system. Therefore, the imaging suites had to be located on elevated floors. A stiff 3-foot-deep steel girder system was utilized to provide a steady platform for the MRIs. This system was ultimately performance verified to provide performance up to 500 m-in/sec to allow for future ultra-high-performance MRIs.
Summary
After 12 years of design, review by the California Office of Statewide Health Planning and Development (OSPHD), Peer Review, and construction, the state-of-the-art hospital opened its doors in October 2019. The base-isolated hospital represents the latest in hospital seismic performance.■
Project Team:
Owner: Stanford Health Care
Structural Engineer: Nabih Youssef Associates
Architect: Rafael Viñoly Architects
Contractor: Clark Construction and McCarthy Building Companies Joint Venture
Base Isolator Supplier: Earthquake Protection Systems
Moat Cover Supplier: Construction Specialties