Advancing First-Generation PBSD for Steel Buildings
Part 2: Case Studies
Implementing performance-based seismic design (PBSD) procedures for assessing existing buildings has generated interest in using similar approaches to design new buildings. The advantage of using these procedures is that designers can go outside the more prescriptive requirements of traditional design and have a more direct connection between expected performance and the design process (i.e., performance targets are explicitly defined upfront). This results in the engineer easily communicating the anticipated performance to the client and targeting a design that achieves beyond-code performance if desired. However, as PBSD was gaining popularity in practice approximately a decade ago, there had been limited published information into the relationship between standards for seismic design of new buildings and the seismic assessment of existing buildings.
As a result, some engineers were concerned that the existing building standard was too conservative, potentially leading to unnecessarily expensive retrofits for existing buildings or unnecessarily expensive designs for new buildings when utilizing the existing building standard for new building design. The need to understand this relationship was noted in the Research Required to Support Full Implementation of Performance-Based Seismic Design (NIST 2009) and in the Perspectives on ASCE 41 or Seismic Rehabilitation of Buildings (SEAONC 2010). Therefore, the National Institute of Standards and Technology (NIST) began a research initiative to help bridge the gap in understanding and address the perceived challenges of adopting PBSD to assess existing buildings and designing new buildings.
Part 1 of this series (STRUCTURE, October 2021) discusses the pertinent history of performance-based design procedures and draws comparisons between performance-based approaches and traditional design approaches. The four-part NIST study, Assessment of First Generation Performance-Based Seismic Design Methods for New Steel Buildings, investigated four steel seismic force-resisting systems (SFRSs) (Harris 2015a, 2015b, 2015c, and Speicher, 2020). Several archetype buildings were designed using the American Society of Civil Engineers’ ASCE 7: Minimum Design Loads for Buildings and Other Structures and then assessed using the provisions in ASCE 41: Seismic Evaluation and Retrofit of Existing Buildings. The results indicated that, in many cases, a building designed to meet the requirements of ASCE 7 did not pass the acceptance criteria in ASCE 41, thus suggesting there is a need for further refinement of PBSD provisions in ASCE 41 to align with a more commonsense outcome.
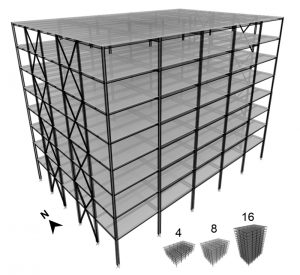
Archetype Buildings
Twenty-four different archetype buildings were designed as part of the NIST study. The buildings were simple in plan layout and framing details to focus on the relationship between new building design and existing building assessment without adding other complexities. Four different SFRS typologies were investigated: special moment frames (SMFs), special concentrically braced frames (SCBFs), eccentrically braced frames (EBFs), and buckling-restrained brace frames (BRBFs). Also, three different heights were investigated: 4-story, 8-story, and 16-story. The moment frames span three bays in the East-West direction, and the braced frames span two bays of the North-South direction. The designs were created using loads from ASCE/SEI 7-10. Each SFRS and height combination was designed twice, using loads via the equivalent lateral force (ELF) procedure and using loads via the modal response spectrum analysis (RSA) procedure. A three-dimensional schematic of the 8-story building is shown in Figure 1.
Building Performance
Once the designs were completed, each building was assessed using the following four levels of Tier 3 assessment procedures in ASCE 41: linear static, linear dynamic (modal RSA), nonlinear static (pushover), and nonlinear dynamic (response history analysis). These four procedures were used to understand the range of assessment outcomes and see how the assessments related to each other. For the linear and nonlinear procedures, models were created in Computer and Structures, Inc’s (CSI) ETABS and Perform-3D, respectively; full details of modeling approaches are documented in the NIST reports. Each assessment was done considering Life Safety (LS) at the Basic Safety Earthquake Hazard Level 1 (BSE-1) (equivalent to the design level earthquake) and Collapse Prevention (CP) at the BSE-2 Hazard Level (equivalent to the maximum considered earthquake). The assessment of the first three system typologies (SMFs, SCBFs, and EBFs) was done using ASCE/SEI 41-06, and the assessment of the BRBFs was done using ASCE/SEI 41-13; different versions of ASCE 41 were used due to the timing of the different phases of the NIST study.
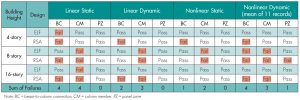
A summary of the SMF assessment outcome is given in the Table. Failures were detected in every level of the assessments conducted, though there was not complete consistency between the linear and nonlinear approaches. For both the linear and nonlinear assessment procedures, deficiencies were detected in the beam-to-column connections and column members. Interestingly, the nonlinear dynamic procedure indicates a few more connection deficiencies than both the linear dynamic and the nonlinear static procedures. This trend counters the idea that the nonlinear dynamic procedure should be the least conservative of the assessment procedures. Similar trends to what was described above were observed for the three different braced frames systems.
Assessment Results
Several trends were identified from the assessment results. In general, assessment using ASCE 41 indicated that a new building design is deficient, especially when utilizing both linear static and nonlinear dynamic procedures. The conservative results seen in the linear static procedures may be considered reasonable given the relatively simplistic methodology utilized to account for what is, in reality, complex nonlinear behavior. In contrast, the nonlinear dynamic procedure should arguably have less conservatism, given that the analysis directly accounts for the nonlinear behavior. It is helpful to probe a few issues to understand the reasons why the nonlinear dynamic procedure gave conservative results, including a) the methodology used to select and scale ground motion records, b) the methodology used to derive acceptance criteria (e.g., ASCE 41 permissible rotations of a beam hinge), and c) the potential that the designs did not actually meet the performance intent of ASCE 7.
The effects of the methodology used for the selection and scaling of ground motion records was explored in Speicher and Harris (2016) and Uribe et al. (2019). The ground motion selection and scaling methodology followed the provisions of Chapter 16 of ASCE/SEI 7-10, with a few exceptions, including using only records selected from the far-field set (i.e., recorded at sites greater than or equal to 10 km from fault rupture) in Federal Emergency Management Agency’s (FEMA) P695: Quantification of Building Seismic Performance Factors (FEMA 2009). This far-field set was compiled for assessing the validity of ASCE 7 seismic performance factors (i.e., response modification factor, R, deflection amplification factor, Cd, and overstrength factor, Ω0), which may result in a ground motion set that is overly demanding for use in an ASCE 41 assessment. Since the intent of the NIST study was to investigate generic archetype buildings, it was reasoned that the FEMA P695 far-field set was an appropriate sampling bin. However, after the results from the NIST study showed that nonlinear dynamic procedures yielded conservative results, this approach was re-examined. Uribe et al. (2019) investigated the effects of using a more hazard-consistent ground motion selection and scaling approach, such as the conditional mean spectrum method. The conservatism was reduced using such a method, but not significantly enough to enable all the building components to pass the nonlinear dynamic assessment.
The next issue explored was related to ASCE 41 acceptance criteria. These criteria are typically derived from available experimental data coupled with supplemental analytical data and engineering judgment. Most experimental data come from steadily increasing fully-reversed cyclic tests such as the standard protocol described in Chapter K of the American Institute of Steel Construction’s (AISC) 341-16. These test protocols demonstrate a component’s behavior under intensive seismic loading. However, it is well-known that a building component’s behavior is often significantly affected by the loading history; the maximum deformations achieved under fully-reversed cyclic loading protocol can be much less than those under a monotonic loading protocol. Most ASCE 41 acceptance criteria found their origins when nonlinear static (pushover) analysis was the state of practice when advanced analysis was employed. At the time, it was logical to have test data that implicitly capture cyclic effects in the backbone curve, which, in turn, is used to define component acceptance criteria. However, the state of practice has changed in the last few decades with the advances in computing power and the general use of nonlinear dynamic analysis becoming more prevalent. Therefore, if the response of a building component does not experience fully-reversed cyclic demands to the degree that capacities were derived, the results are often conservative, sometimes to a great degree. This observation is combined with the trend of benchmarking building performance to collapse likelihood. However, several studies have highlighted the tendency for a building to have a one-side response with ratcheting behavior when subject to collapse level shaking (Ibarra and Krawinkler 2005, Lignos and Krawinkler 2011, Maison and Speicher 2016, Speicher and Harris 2016). Therefore, having acceptance criteria that adapt based on loading history would be logical and advantageous. Still, the implementation of such criteria is challenging given the complex failure mechanisms of building components and the limited availability of tests utilizing alternative loading protocols to validate such new criteria.
Some ASCE 41 component criteria are used as surrogates to capture other phenomena not captured in typical nonlinear models. Therefore, the challenge remains on how to best address a component’s performance considering loading history. One possible approach is to generate assessment criteria dependent upon loading history, such as energy-based acceptance criteria for certain components.
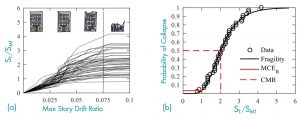
The third issue explored was the potential that the archetype building designs do not actually achieve the intent of ASCE 7, which is specified as less than or equal to a 10% probability of collapse given a risk-targeted maximum considered earthquake (MCER). If the designs do not meet this goal, then ASCE 41 would be justified in flagging the buildings as deficient. Therefore, to validate the designs, NIST conducted additional studies into the seismic performance of the SMFs utilizing the methodology from FEMA P695. New two-dimensional models were generated using OpenSees (the Open System for Earthquake Engineering Simulation) and were compared to the Perform-3D models to verify their consistency. OpenSees is an object-oriented software framework created at the National Science Foundation-sponsored Pacific Earthquake Engineering Research (PEER) Center. Incremental dynamic analysis “spaghetti” curves were generated as shown in Figure 2a, and the associated fragility curve is shown in Figure 2b for the 8-story ELF-designed SMF. Value ST is the median spectral acceleration of the record set at the fundamental period of the building, SMT is the value of the MCER at the fundamental period of the building, and CMR is the collapse margin ratio defined as the ST/SMT value where 50% of the ground motion records result in a collapse of the building. The spaghetti curves are generated by incrementally scaling an individual ground motion record and recording the associated maximum interstory drift from the building response. The fragility curve is the cumulative distribution function of the collapse levels obtained from the spaghetti curve results.
For the 8-story ELF-designed SMF, the collapse margin ratio is approximately 2.0. This means the entire record set must be scaled by 2.0 before half the records cause collapse. The FEMA P695 methodology further requires the CMR to be adjusted considering the spectral shape of the ground motions. Thus the adjusted collapse margin ratio turns out to be approximately 3.22 for this example. The acceptable collapse margin ratio considering a 10% probability of collapse and a total system uncertainty of 0.53 is 1.96. Therefore, the 8-story ELF-designed SMF has a margin against collapse of approximately 1.64 (= 3.22/1.96) times greater than required to satisfy the 10% conditional goal. A full explanation of the results can be found in Collapse Risk of Steel Special Moment Frames per FEMA P695 (Speicher et al., 2020). Ultimately, the results indicate that the SMFs satisfy the 10% objective, and therefore indicate that the ASCE 41 provisions utilized provide an overly conservative result. Work is ongoing at NIST to investigate the collapse probability of the archetype buildings with the other three SFRSs.
PBSD research at NIST has also expanded to several other projects to support further advancement and implementation. For example, given the results from volumes 1-3 published in 2015, NIST sponsored related research to advance the state of practice for PBSD, which resulted in the report titled Recommended Modeling Parameters and Acceptance Criteria for Nonlinear Analysis in Support of Seismic Evaluation, Retrofit, and Design (NIST 2017). This report made recommendations for broad improvements to seismic nonlinear modeling and acceptance criteria requirements for various structural systems. NIST also sponsored an extensive experimental investigation looking at the performance of deep wide-flange steel members, which are often used in special moment frames. The results of this research are published in Seismic Behavior and Design of Deep, Slender Wide-Flange Structural Steel Beam-Columns (NIST 2021). Several recommendations from these reports were considered in the ASCE/SEI 41-17 update cycle and are currently being considered for the ASCE/SEI 41-23 update cycle.
Conclusions
A set of steel buildings were designed with the loads specified in ASCE/SEI 7-10 and then assessed with ASCE/SEI 41-06 or ASCE/SEI 41-13. Four levels of analysis were conducted as part of an ASCE 41 Tier 3 assessment. In general, the results indicated that the steel buildings studied have deficiencies that would need to be retrofitted to satisfy ASCE 41. These results are contingent on the choices made during the design and assessment process, some of which were further critiqued to shed light on how the ASCE 41 assessment can be improved so that a more logical outcome can be achieved. Of specific note was the seemingly overly conservative results from the nonlinear dynamic procedure. The methodology utilized for selecting and scaling ground motions was shown to add to the conservative outcome. However, even with changes to the selection and scaling approach, building designs still failed the ASCE 41 nonlinear dynamic assessment.
Other issues related to the accounting of loading history in the acceptance criteria were also explored in the NIST study. ASCE 41 component acceptance criteria are derived from a combination of fully-reversed cyclic tests and engineering judgment. However, it is well-known that component behavior does not necessarily follow a fully-reversed loading pattern during an earthquake, especially when subjected to near-fault collapse level shaking. Currently, the acceptance criteria do not account for the differences based on loading history, which can add a layer of conservatism to the results. Suggestions have been proposed on how this may be addressed, but the complexities remain. For example, the intent of engineering judgment and how a component may be a surrogate for other behavior is challenging to quantify.
The final issue discussed is the notion that the archetype building design may not meet the intended performance goal of ASCE 7; thus, as a corollary, the ASCE 41 assessment rightly flags the designs as deficient. An investigation using FEMA P695 was conducted to test this idea which showed that the designs were, in fact, satisfactory. This confirms the conservatism in the ASCE 41 procedures, given all the choices and assumptions made to arrive at these conclusions. It is particularly interesting when using ASCE 41 as an alternative approach to designing new buildings. The conservatism seems to take some motivation away from utilizing such PBSD procedures for new designs. The results suggest several areas need improvement in ASCE 41 if alignment with ASCE 7 is desired. Therefore, additional research funded by NIST has further expanded the PBSD efforts to update modeling and acceptance criteria based on new experimental data and state-of-the-art research.
Part 3 will discuss the future of PBSD in practice, including its relationship to resilience-based design, which aims to quantitatively support community resilience.■
References
FEMA (2009). Quantification of Building Seismic Performance Factors. FEMA P695. Federal Emergency Management Agency. Washington, D.C.
Harris, JL, Speicher, MS (2015). Assessment of First Generation Performance-Based Seismic Design Methods for New Steel Buildings Volume 1: Special Moment Frames, NIST TN 1863-1, National Institute of Standards and Technology, Gaithersburg, MD. February 2015. http://dx.doi.org/10.6028/NIST.TN.1863-1
Harris, JL, Speicher, MS (2015). Assessment of First Generation Performance-Based Seismic Design Methods for New Steel Buildings Volume 2: Special Concentrically Braced Frames, NIST TN 1863-2, National Institute of Standards and Technology, Gaithersburg, MD. http://dx.doi.org/10.6028/NIST.TN.1863-2
Harris, JL, Speicher, MS (2015). Assessment of First Generation Performance-Based Seismic Design Methods for New Steel Buildings Volume 3: Eccentrically Braced Frames, NIST TN 1863-3, National Institute of Standards and Technology, Gaithersburg, MD. http://dx.doi.org/10.6028/NIST.TN.1863-3
Ibarra, LF, and Krawinkler, H (2005). Global Collapse of Frame Structures under Seismic Excitations, Rep. No. 152, Pacific Earthquake Engineering Research Center, Berkeley, CA.
Lignos, DG, and Krawinkler, H (2011). Prediction and validation of sidesway collapse of two scale models of a 4-story steel moment frame. Earthquake Engineering and Structural Dynamics, 40:807-825. https://doi.org/ 10.1002/eqe.1061
Maison, B. F., Speicher, M. S., 2016. Loading Protocols for ASCE 41 Backbone Curves. Earthquake Spectra 32(4), 1-20. https://doi.org/10.1193/010816EQS007EP
NIST (2009). Research Required to Support Full Implementation of Performance-Based Seismic Design. NIST GCR 09-917-2. National Institute of Standards and Technology, Gaithersburg, MD.
NIST (2017). Recommended Modeling Parameters and Acceptance Criteria for Nonlinear Analysis in Support of Seismic Evaluation, Retrofit, and Design. NIST GCR 17-917-45. National Institute of Standards and Technology. Gaithersburg, MD.
SEAONC (Structural Engineers Association of Northern California), Existing Buildings Committee, Perspectives on ASCE 41 or Seismic Rehabilitation of Buildings, STRUCTURE magazine, October 2010.
Speicher, MS, Dukes, JD, Wong, KF (2020). Collapse Risk of Steel Special Moment Frames per FEMA P695. NIST TN 2084. National Institute of Standards and Technology. Gaithersburg, MD.
Speicher, MS, Harris, JL (2016). Collapse Prevention Seismic Performance Assessment of New Eccentrically Braced Frames using ASCE 41, Engineering Structures, 117, 344-357. https://doi.org/10.1016/j.engstruct.2016.02.018
Speicher, MS, Harris, JL (2020). Assessment of First Generation Performance-Based Seismic Design Methods for New Steel Buildings, Volume 4: Buckling-Restrained Braced Frames. NIST TN 1863-4. National Institute of Standards and Technology. Gaithersburg, MD. https://tsapps.nist.gov/publication/get_pdf.cfm?pub_id=922024
Uribe, R, Sattar, S, Speicher, MS (2019). Effect of Common U.S. Ground Motion Selection Methods on the Structural Response of Steel Moment Frame Buildings, Earthquake Spectra, 35(4), 1611-1635, https://doi.org/10.1193/122917EQS268M