Preventing Heat Loss Through Concrete Parapets
Conventional concrete parapets can form a thermal bridge that dissipates heat energy outside like large cooling fins, aided by cold winds whipping over the rooftop. One solution is to cast a structural thermal break between the parapet and the heated interior structure supporting it.
Parapets protect the edge of roof assemblies from uplift forces created by winds blowing against and over the building. However, solving one problem creates another: a thermal bridge the length of the building perimeter that penetrates the insulated building envelope, conducting heat energy from the building’s interior support structure and dissipating it into the environment. In addition to energy waste, uninsulated parapets chill the heated interior space adjacent to the building envelope, creating uncomfortable living spaces because of the non-uniform temperature distribution.
The traditional approach to mitigating thermal bridging at parapets is to wrap its exposed surfaces with insulation, making it a part of the heated building mass.
A newer method addresses thermal bridging more effectively: thermally separating or “breaking” the parapet from the heated interior structure by casting structural thermal break modules between the two masses. These thermal breaks are optimized for thermal performance without affecting structural integrity.
Thermal Bridging – a Primer
“Thermal bridging in building construction occurs when thermally conductive materials penetrate the insulation, creating areas of reduced resistance to heat transfer. These thermal bridges are most often caused by structural elements that transfer loads from the building envelope back to the building superstructure” (Payette Architects 2015). The results of thermal bridging include higher heat transfer resulting in colder internal surface temperatures, higher energy use for heating and cooling, noncompliance with building regulations, building occupant discomfort, and potential for condensation and mold.
The two most common types of thermal bridging are material and geometric (Figure 1). When an element made from a material of high thermal conductivity penetrates an insulating layer, and the protrusion conducts heat at a higher rate than does the insulation, a material thermal bridge is created. Examples include balconies, as conductive elements that penetrate the vertical building envelope, and parapets, as elements that penetrate the roof insulation.
Geometric thermal bridging occurs when a heat-emitting surface is larger than the heat-absorbing surface. Building corners are a typical example, but geometric thermal bridging can also affect wall/roof and wall/floor junctions, junctions between windows, walls, and doors, and, of course, parapets. Most of the time, material and geometric thermal bridging occur in concert.
Heat transmittance through thermal bridges can be idealized as linear or point:
- Linear transmittance through thermal bridges occurs with disturbances in the continuity of the thermal envelope along a certain length. Typical examples include concrete balcony connections with the floor slab penetrating the wall, outer wall edges, floor supports, and window-to-wall junctions.
- Point transmittance through thermal bridges occurs with disturbances in one spot that penetrate an insulating layer. Examples include steel balconies, canopies, and roof extensions.
While heat energy loss is an obvious consequence of thermal bridging, developers are faced with a newer and potentially more significant outcome: condensation and mold growth.
Since older buildings often leaked air profusely, interior humidity levels equalized with low exterior humidity levels, typically between 18% to 25% during winter months. Forced hot air blowing at or near cold penetrations, such as balconies and parapets, further ensured that the local interior humidity never rose to the dew point, thus preventing condensation and mold growth.
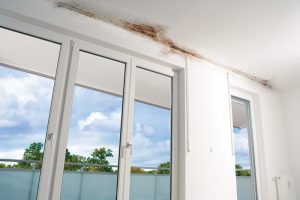
Figure 2. Condensation on the chilled interior side of uninsulated penetrations supports the growth of mold, which can become airborne years before spreading to visible wall and ceiling surfaces.
Because modern buildings are airtight, humidity levels can reach 30% to 40% during winter months. While comfortable for occupants, high interior humidity near chilled building penetrations can reach the dew point, forming condensation that supports mold growth on adjacent surfaces such as sheetrock, insulation, and any cellulose-based material, particularly in stagnant air cavities (Figure 2). Mold can become airborne years before it migrates to visible surfaces, exposing the developer to potential liability and remediation costs.
A Closer Look at Parapets
Parapets play an important architectural and structural role in buildings. However, being at a sensitive location – at the connection of the roof and wall envelope – they are subject to various potential performance issues. In his paper, Parapets: Where Roofs Meet Walls, Joseph W. Lstiburek, Ph.D., mused, “Historically, so many problems have occurred with parapets that we have a name for it: parapetitus. Thermal bridging is everywhere.”
As a high-mass structural element that penetrates the building at its windiest point, parapets are especially susceptible to thermal bridging and its consequences.
Wrapping Exterior Parapet Surfaces
To prevent thermal bridging, architects historically wrapped the perimeter of the wall with an insulation barrier and then wrapped the parapet as well, making it part of the heated building mass (Figure 3). In addition to being costly and minimally effective, this method presented long-term risks.
![Figure 3. Left: Continuous parapet connections without thermal break. Right: Parapet connection was thermally broken with a structural thermal break. Courtesy of Schock Isokorb Design Guide [5752].pdf.](https://www.structuremag.org/wp-content/uploads/2020/08/0920-sd-3-300x138.jpg)
Figure 3. Left: Continuous parapet connections without thermal break. Right: Parapet connection was thermally broken with a structural thermal break. Courtesy of Schock Isokorb Design Guide [5752].pdf.
Designers at Payette researched this subject in their article (Payette 2015): “One question we found intriguing was whether it was better to insulate around the parapet – covering all structural interfaces – or underneath it by finding a way to design a structural connection that effectively attaches the parapet after installation of the insulation. Since there are many variables in the detailing of a parapet, we started with a sensitivity analysis of parapet height normalized to one construction type. Because the degree of impact of the assembly depends on how much of the building we are looking at in conjunction with the parapet, we also normalized on an extracted detail that includes 24 inches (61 cm) in height of inside wall surface and 48 inches (122 cm) in length of inside roof surface.”
The Payette findings were compelling but not surprising. Naturally, the higher the wrapped parapet, the greater the potential for energy loss. Payette also discovered that insulating beneath the parapet – thermally breaking it from the roof – negated the height factor and provided the most effective insulation, even though there was no thermal break specifically designed for parapets at the time. “We tested a commercially available structural thermal break designed for concrete slabs (balconies) and installed this in a vertical orientation. The improvement decreased the heat flow through the assembly by 27%.”
New Parapet Paradigm
Today, manufactured structural thermal breaks designed explicitly for parapets are commercially available. They thermally isolate the parapet from the heated interior, while preserving the structural integrity of the connection.
A typical manufactured structural thermal break for parapets (Figure 4) is a fabricated assembly consisting of enhanced insulation and stainless steel reinforcing bars, creating a module capable of transferring the loads from the parapet to the concrete roof slab that supports it, while minimizing thermal conductivity between the two concrete masses (Figure 5). The insulating block, made of graphite-enhanced expanded polystyrene, is roughly 98% less conductive than concrete, and the stainless steel reinforcing bars are approximately 70% less conductive than carbon steel reinforcing bars, effectively reducing heat loss at the penetration by up to 90%.
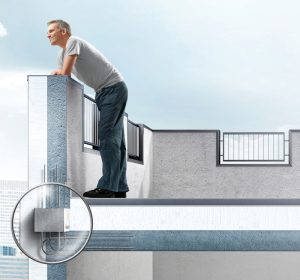
Figure 5. Rendering of a structural thermal break cast between a concrete roof slab and concrete parapet.
The vertical reinforcing bars resist tension/compression and bending moments on the parapet. The interaction between applied bending moments and tension/compression is taken into consideration. The crossed bars provide the necessary shear strength, in either direction, through the tensile strength of these bars.
Other Concrete-to-Concrete Construction
In addition to parapets, thermal bridging can occur at balconies when constructed conventionally as structural extensions of interior floor slabs. In cold weather, the balcony conducts heat energy from the heated interior floor slab, dissipating it into the environment while chilling the interior floor opposite the balcony.
Structural thermal breaks designed for balconies are comprised of insulating blocks the same approximate width of the building wall. Stainless steel reinforcing bars are cast into the concrete slab on the interior side and the concrete balcony on the exterior side, providing the necessary structural strength and stiffness at the connection, working as a truss system.
Similar structural thermal breaks are available for the insulation of slab edges.
Costs vs. Benefits
While the manufactured structural thermal breaks come with a higher initial investment, there are benefits in the short- and long-term, which compensate such costs.
Maintenance-free, once installed, the module reportedly simplifies the formwork process, reducing construction costs versus the conventional parapet insulation wrapping method.
Compared to wrappings, it eliminates costs from future restoration related to water penetration (Figure 6).
Compared to non-insulated connections, the addition of structural thermal break elements can achieve a significant reduction in thermal conductivity at the connection area for standard load-bearing scenarios, typically 50 to 90%, translating into overall reductions in energy use for the building.
The reduction in energy to heat the building also allows corresponding reductions in heating system size/capacity, resulting in additional savings on capital equipment and ongoing operation and maintenance of mechanical systems.
Thermal isolation of balconies and slab edges also improves comfort for occupants and provides value for developers by increasing the warmth and usability of interior floor space.
In addition to these tangible values, structural thermal breaks can prove instrumental in complying with tightening building codes that mandate higher energy efficiency and continuous insulation.■
Parapet Thermal Breaks Help Attain LEED Gold
A major developer in British Columbia, Canada, has been an early adopter of thermal breaks for balconies and shading eyebrows in its Vancouver senior residence projects. Following these successful experiences, the developer employed a similar solution for a subsequent Vancouver project that included parapets in addition to balconies and eyebrows. In part, it helped meet the new LEED Gold certification requirements for new construction.
For the 23-story tower main building and a two-story auxiliary building, 199 residential units and the tower building incorporated 1,820 concrete-to-concrete thermal breaks that insulate 5,970 linear feet (1,820m) of balconies from the interior.
Thermally broken parapets form the perimeter of levels two and three in both the main tower and the auxiliary building, for a total 1,100 feet (335.3m).
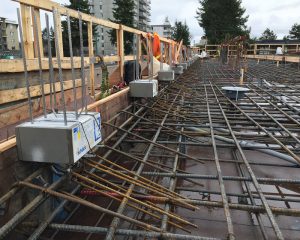
Figure 7. Structural thermal breaks positioned against wooden parapet forms are tied into rooftop reinforcement before casting in concrete.
This was the first time the company employed structural thermal breaks for parapets. The construction managers reported, “We’re always looking to improve on the comfort, efficiency, and sustainability of our facilities. So, this was the perfect setting for us to work on incorporating the thermal breaks for parapets. If you’re going to be an owner/developer and your facility has parapets, this thermal break concept has significant benefit.” (Figure 7)
References
Schöck Isokorb® Type CPA for Concrete Parapets, Schöck North America
Parapets: Where Roofs Meet Walls, (June 2011) Building Science Corporation
Thermal Bridging Research: Parapets (Payette Architects, July 2015)
Schöck Thermal Bridging Guide, (May 2018), Schöck Ltd. (UK)
Facts about Mold and Dampness, US Centers for Disease Control and Prevention
Room Climatic Conditions in Residential Areas, Heilman, K et al. (1999)