The rise in performance-based engineering, in which a structure is proportioned to meet certain predictable performance requirements, necessitates reasonable estimates of component behavior during earthquakes. It is customary to determine component properties via physical lab tests. For components such as concrete anchors, verification of the ultimate strength is required and quasi-static pull tests are sufficient. The situation is more involved for other components, such as beam-to-column assemblies, since an earthquake produces dynamic back-and-forth cyclic actions and the component is often expected to deform inelastically.
Although earthquake loadings are dynamic, quasi-static component tests are routinely performed due to the complexity and expense of dynamic testing (Figure 1). The most widely used loading patterns (protocols) consist of fully-reversed cyclic loading having progressively increasing displacement amplitudes. These are often referred to as simply “cyclic” tests and are termed here as “standard” protocols.
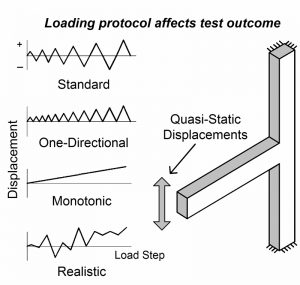
Figure 1. Illustration for a lab test of beam-to-column assembly and various quasi-static loading protocols.
Figure 2 contrasts a standard protocol to that of a simulated earthquake response from a building undergoing inelastic actions. Notice the standard protocol has many cycles as opposed to the earthquake response having relatively few cycles with a one-direction bias. The Applied Technology Council project ATC-62 was one of the first extensive studies that found standard protocols too demanding compared with actual earthquake loadings, and the use of standard protocols can lead to the overly-conservative representation of component seismic performance (FEMA, 2009).
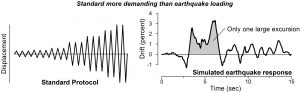
Figure 2. Typical standard protocol compared to simulated building inter-story drift earthquake response (Maison et al., 2016)
Reports on lab tests often do not discuss how a component response is influenced by the loading protocol, so engineers are left to accept the results as intrinsic “seismic” properties. This behind-the-scenes aspect is the subject of this article. The purposes are to:
- Illustrate differences in test results from using standard protocols and actual earthquake loading patterns.
- Point out that lab test data based solely on standard protocols can lead to unduly conservative component acceptance criteria as well as computer models that over-estimate building response in performance-based engineering.
- Encourage future experimental projects to use protocols reflecting actual earthquake response patterns to better estimate component seismic behaviors.
Realistic Protocols
With the advancement and gaining popularity of nonlinear structural analysis, a better understanding of actual seismic response has led researchers to propose different protocols that may more appropriately reflect earthquake inelastic building response (Figure 3). Such protocols are termed here as “realistic.” Realistic protocols are different from standard protocols by having fewer cycles and a one-direction bias.
Tests using realistic protocols have been relatively infrequent, but they do show that components generally have more ductility compared to results using standard protocols. For example, Figure 4 shows the drifts describing various damage states of steel columns from tests conducted by Elkady et al (2018). Use of a realistic collapse-consistent protocol indicates the columns have about twice the inelastic deformation capacity than those from using standard protocols. Accordingly, column acceptance criteria would be very conservative should they be based entirely on the standard protocol test results.
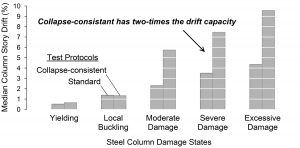
Figure 4. Drifts defining column damage states from lab tests using different loading protocols (Elkady et al., 2018).
Backbone Curves
In performance-based engineering, the backbone curve is the customary way of describing component behaviors over a range of deformations. It is formulated as an envelope of hysteresis loops from component lab tests and is the chief factor for displacement-controlled component modeling and acceptance criteria.
Figure 5 illustrates the strong influence the protocol can have on envelopes (backbones) of test results from identical reinforced concrete bridge piers (FEMA, 2009). The backbones were essentially the same out to about 2% drift, but they differed significantly for larger drifts depending on the protocol. Notice how the standard protocols produced backbones with the smallest drift capacities.
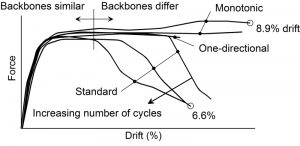
Figure 5. Envelopes of cyclic test results (backbones) from six identical reinforced concrete bridge piers subjected to various loading protocols (adapted from Figure 2-20 of the ATC-62 project; FEMA P-440A).
The trend is also evident in other materials. Figure 6 shows results from identical plywood shear walls subjected to different protocols from a test conducted by Gatto and Uang (2003). There was little difference between the cyclic envelope and the monotonic test out to about a 3% drift. However, at about 4% drift, the cyclic envelope strength was less than one-half that of the monotonic test strength.
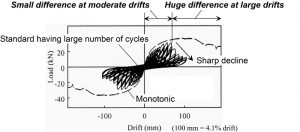
Figure 6. Lab test results from tests of two identical plywood shear walls using different loading protocols. (Adapted from Figure 6g in Gatto et al., 2003)
Figure 7 shows more test results from identical plywood shear walls but, in this case, a more realistic protocol is compared to the monotonic. The envelope from the realistic test is similar to the monotonic test suggesting that earthquake inelastic behavior is better represented by the monotonic as opposed to the standard protocol. In fact, Professor Helmut Krawinkler, who was responsible for the development of several popular loading protocols, advocated complementing standard tests with other tests, including monotonic, whose loading histories better represent response close to collapse (Krawinkler, 2009).
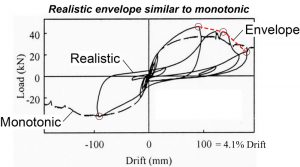
Figure 7. Lab test results from two identical plywood shear walls using different loading protocols. (Adapted from Figure 6h; Gatto et al., 2003)
Therefore, key points about backbone curves derived from standard lab tests are as follows:
- Standard protocols do not mimic actual earthquake demands. Lab tests using such protocols generally result in backbones having progressively decreasing deformation capacities according to increasing numbers of fully-reversed loading cycles.
- For moderate drifts, say up to about 2%, backbone curves are generally independent of the loading protocol.
- For large drifts, say greater than about 2%, backbone curves can be strongly influenced by the loading protocol.
Additional Studies
The National Institute of Standards and Technology (NIST) performed comprehensive analytical investigations into the correlation between the seismic performance of steel buildings designed to current standards and their performance as quantified using performance-based engineering (Harris and Speicher, 2015). It was found that performance-based engineering often rejects “new” buildings as being unsafe even though they meet current building codes. One likely reason is conservatism in performance-based engineering acceptance and modeling criteria since the vast majority of existing lab test data used standard protocols having the shortcomings discussed above (Speicher et. al, 2018).
Likewise, the ongoing Applied Technology Council project ATC-116 investigates the paradox of the better-than-expected performance of short period buildings. One principal finding is the important effect of the component post-peak residual strength (Kircher et al., 2018). To arrive at performance observed in actual earthquakes and to be consistent with the judgment of earthquake engineers, the ATC-116-improved analytical models have significant residual strength beyond 10% drift (30% to 60% of component ultimate strength). Lab tests using realistic protocols generally have much greater residual strength than those using standard protocols (Figures 6 and 7). This gives support to the notion that lab tests using standard protocols lead to conservative seismic performance criteria (Speicher et al, 2018).
ASCE 41 Performance-Based Engineering
ASCE 41, Seismic Evaluation and Retrofit of Existing Buildings, is an industry standard incorporating performance-based engineering methods (ASCE, 2017). Figure 8 depicts the ASCE 41 construction of a backbone curve as an envelope of data from a component lab test using a standard protocol. The peak displacement reversal points in the hysteresis loops govern where the abrupt decline in strength occurs in the backbone, giving the impression that the component has zero strength beyond deformation E. However, this is misleading since the use of a protocol having different displacement reversal points would shift the deformation to E´ as indicated. Hence, the backbone curve is dependent on the loading protocol, and standard protocols do not reflect building seismic inelastic response.
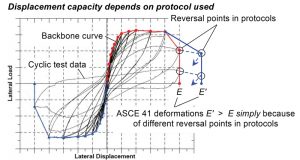
Figure 8. Construction of ASCE 41 component backbone curves as envelopes of cyclic test data (adapted from Figure 7-5 in previous ASCE 41-13). The displacement reversal point is at E in one protocol, whereas it is at E´ in another protocol.
The latest edition of ASCE 41, ASCE 41-17, recognizes the importance of loading protocols and provides additional freedom in protocol selection to better represent actual seismic loading patterns. It does not prescribe a specific “one-size-fits-all” loading protocol due to the variety of factors involved with a particular component, e.g., performance objective, type of structure, and seismic setting.
Since most previous component tests were performed using standard protocols, ASCE 41-17 allows such test data to be supplemented to better define behavior at near-collapse displacements (Section 7.6). To ensure reasonable protocols are selected for a particular component and project, concurrence is required by independent peer reviewers. The rationale for the current ASCE 41 provisions is in a paper by Maison and Speicher (2016).
Conclusion
With the emergence of performance-based engineering, it is essential to have reasonable estimates of component behaviors during actual earthquakes. Standard lab test loading protocols typically consist of fully-reversed cyclic loading with progressively increasing displacement amplitudes. However, realistic earthquake loading patterns are not like standard protocols and can lead to different conclusions about component performance.
There are two underlying shortcomings when using component lab test data derived from standard protocols.
- Ductility can be underestimated, which, in turn, can lead to overly conservative acceptance criteria.
- Derived backbone curves used in analysis models for seismic evaluation can lead to over-estimation of peak inelastic displacements.
The above shortcomings have a compounding effect, causing rejection of buildings that would otherwise be considered acceptable should component behaviors be based on tests using realistic earthquake loading patterns.
When using lab test data, it is important to be aware of the loading protocol used. The test results must be scrutinized within the context of the loading protocol. If a standard protocol is used, then it is likely the data represents a very conservative description of component inelastic behavior under earthquake loadings. In this case, it may be appropriate to modify the data for use in performance-based engineering. The current ASCE 41-17 outlines one way this can be done. It is encouraged that future lab tests include realistic earthquake loading protocols so that the results are best suited for performance-based engineering.■
References
Federal Emergency Management Agency (FEMA) (2009). The Effects of Strength and Stiffness Degradation on Seismic Response, Technical Report FEMA P-440A (ATC-62 Project), Washington D.C.
Elkady, A., Ghimire, S., and Lignos, D. (2018). Fragility curves for wide-flange steel columns and implications for building-specific earthquake-induced loss assessment, Earthquake Spectra, Earthquake Engineering Research Institute, vol. 34, no. 3, August, 1405-1429.
Gatto, K., and Uang, C-M. (2003). “Effects of loading protocol on the cyclic response of wood frame shearwalls.” Journal of Structural Engineering, ASCE, vol. 129, no. 10, October, pp.1384-1393.
Krawinkler, H., (2009) “Loading histories for cyclic tests in support of performance assessment of structural components.” Proceeding of the 3rd International Conference on Advances in Experimental Structural Engineering (3AESE), San Francisco, October.
Harris, J.L., and Speicher, M.S. (2015). Assessment of First Generation Performance-Based Seismic Design Methods for New Steel Buildings, Volumes 1: Special Moment Frames, National Institute of Standards and Technology (NIST), Gaithersburg, MD. http://dx.doi.org/10.6028/NIST.TN.1863-1.
Speicher, M.S., Dukes, J., and Wong, K.K.F. (2018). Using FEMA P695 to Interpret ASCE 41 Seismic Performance of Special Moment Frames. Proceedings of the 11th National Conference in Earthquake Engineering, Earthquake Engineering Research Institute, Los Angeles, CA.
Kircher, C., Pang, W., Ziaei, E., and Filiatrault, A., and Heintz, J. (2018). “Solutions to the short-period building performance paradox” Proceedings of the 11th National Conference on Earthquake Engineering, Earthquake Engineering Research Institute, Los Angeles, CA.
American Society of Civil Engineers (ASCE) (2017). Seismic Evaluation and Retrofit of Existing Buildings. ASCE Standard ASCE/SEI 41, Reston, VA.
Maison, B.F., and Speicher, M.S. (2016). “Loading protocols for ASCE 41 backbone curves.” Earthquake Spectra, Earthquake Engineering Research Institute, vol. 32, no. 4, November, 2513-2532.
Speicher, M.S. and Maison, B.F., “The Blind Side: Using ‘Canned’ Loading Protocols in Seismic Testing,” Proceedings of the 12th Canadian Conference on Earthquake Engineering, Quebec City, Canada, June 2019.