Electric vehicle weights endangering parking structures.
Converting the U.S. passenger vehicle fleet to electric power is a cornerstone of the national leadership’s proposed response to climate change. The electric vehicle tradeoff of reduced carbon emissions for increased vehicle weight creates a historic opportunity for the engineering and construction industries to adapt infrastructure to support societal resiliency.
While the opportunity is historic, it is not without precedent, and the examples are a call to action by engineers and builders. Recent history provides instances of promising technologies nearly eradicated by early failures. The liberty ships essential for the Allied victory in WWII had early catastrophic failures that only with rapid resolution of welding flaws avoided constriction of the war’s sea lifeline. The 1994 Northridge Earthquake brought a moratorium to welded steel moment frame construction after the temblor revealed the lateral system previously codified as the most ductile system to be brittle. Rapid engineering response produced retrofits of existing frames and developed new joint configurations to make welded steel moment frames reliable as originally believed. Failure to enable the shift to heavier electric vehicles (EVs) may impair one of the most broadly recognized remedial measures for climate change. Engineers and builders have an obligation to provide sound infrastructure for EVs to prevent early catastrophic failures from blighting adoption of the technology.
Obvious infrastructure vulnerable to damage due to increased vehicle weight are passenger vehicle parking garages. While there are other infrastructures, such as bridges and roads, that are also jeopardized by increased vehicle weight, we use parking structures to illuminate the need for action to compensate for the increased electric vehicle weights.
Significantly increasing passenger vehicle weights combined with recently reduced structural design requirements will result in reduced factors of safety and increased maintenance and repair costs for parking structures. The reduced factors of safety will increase the rate of structural deterioration and the frequency of structural failures. Parking structure maintenance and repair costs will increase from heavier wear on traffic coatings, concrete repair of cracking floors and beams, and/or necessary structural strengthening or retrofit of the overall structure. Serviceability will be impaired by increased floor vibrations, increased floor deflections, and visible concrete cracking. These deleterious effects are consequences for code-compliant design and construction; sub-standard parking structures will suffer more.
To develop practical and prudent approaches to mitigate the inexorable increase in passenger vehicle weights, we review the structural effect of the added loads to quantify the risks imposed on parking garage owners, users, and stakeholders. Many existing parking structures need repair, and there is a high probability that they do not meet current or historic code-loading requirements. There are many cases of parking structure failures, and the growing demand for EVs will only increase the probability of failure.
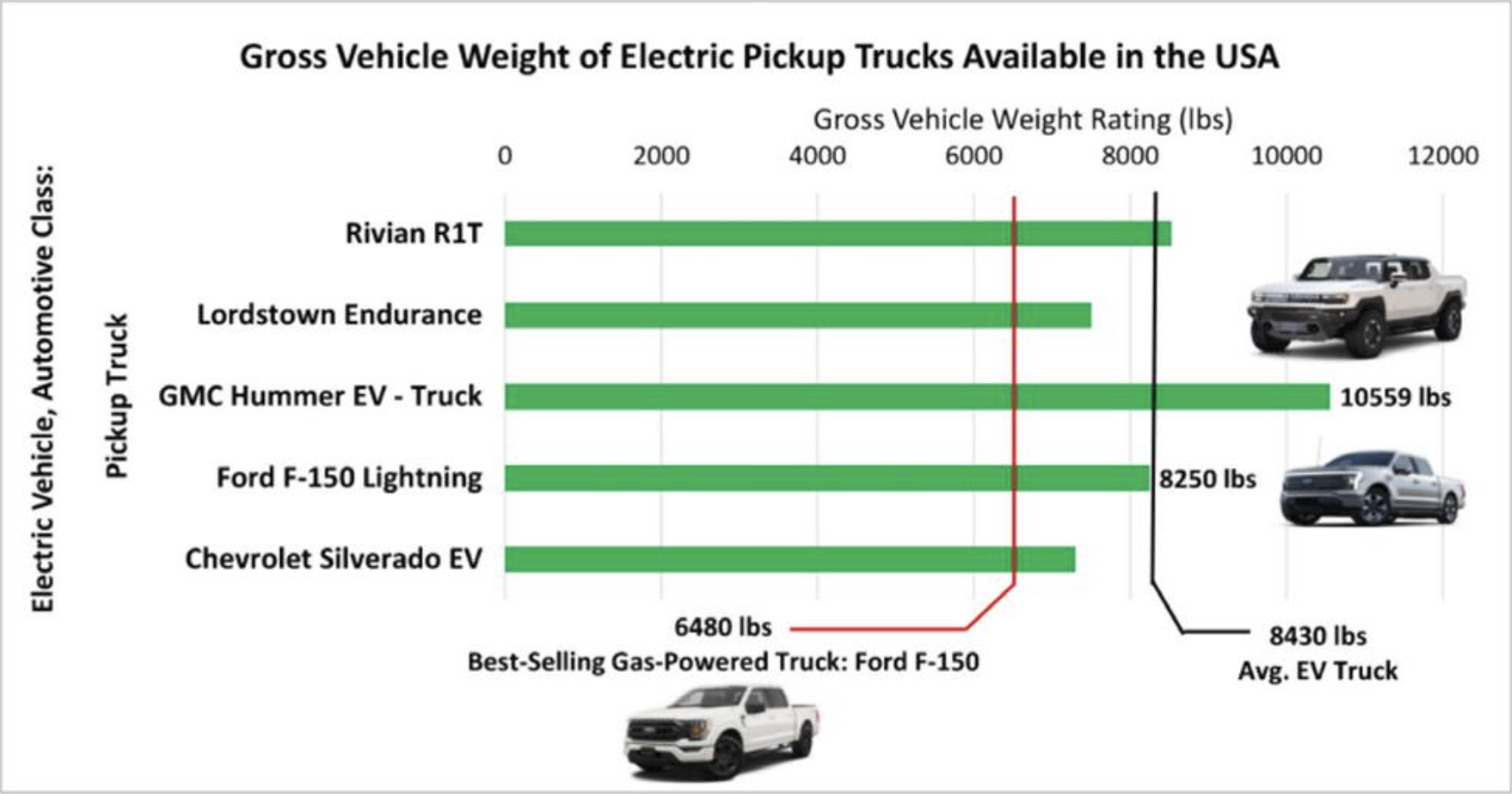
Electric Vehicle Trends
The excitement about sleek, powerful, and futuristic EVs has over-shadowed the fundamental fact that EVs weigh significantly more than an equivalent-sized combustion engine vehicles. While EVs have much smaller and lighter motors than their internal combustion engine (ICE) counterparts’ engines, they typically use heavy lithium-ion batteries that add substantial weight to an EV’s drivetrain. The average combustion engine weighs between 300 and 700 pounds, while the battery for most EVs weighs around 1,000 pounds. In fact, the battery of GMC’s Hummer EV truck weighs nearly 3,000 pounds or the weight of an entire Honda Civic. These battery weights dwarf the gasoline weight (180 pounds) of a full, exceptionally large gas tank, 30 gallons. Figures 1 and 2, based on 2023 manufacturer’s gross vehicle weight rating (GVWR) data, depict how much heavier a sample of EV trucks and EV sedans, respectively, are in comparison to a comparable representative ICE vehicle. The average GVWR of the EV trucks is 8,430 pounds, approximately 2,000 pounds more than the gas-powered Ford F-150, the best-selling truck in the United States. The electric Ford F-150, the Ford F-150 Lightning, weighs over 25% more than the gas-powered Ford F-150 when trucks of an equivalent wheelbase and drivetrain are compared. In addition, the GMC Hummer EV pickup truck weighs over 10,000 pounds, making it currently the heaviest car on the road and over 4,000 pounds more than the Ford F-150. For sedans, the average EV sedan weighs just over 6,000 pounds, 30% more than the U.S.’s most popular sedan, the Toyota Camry.
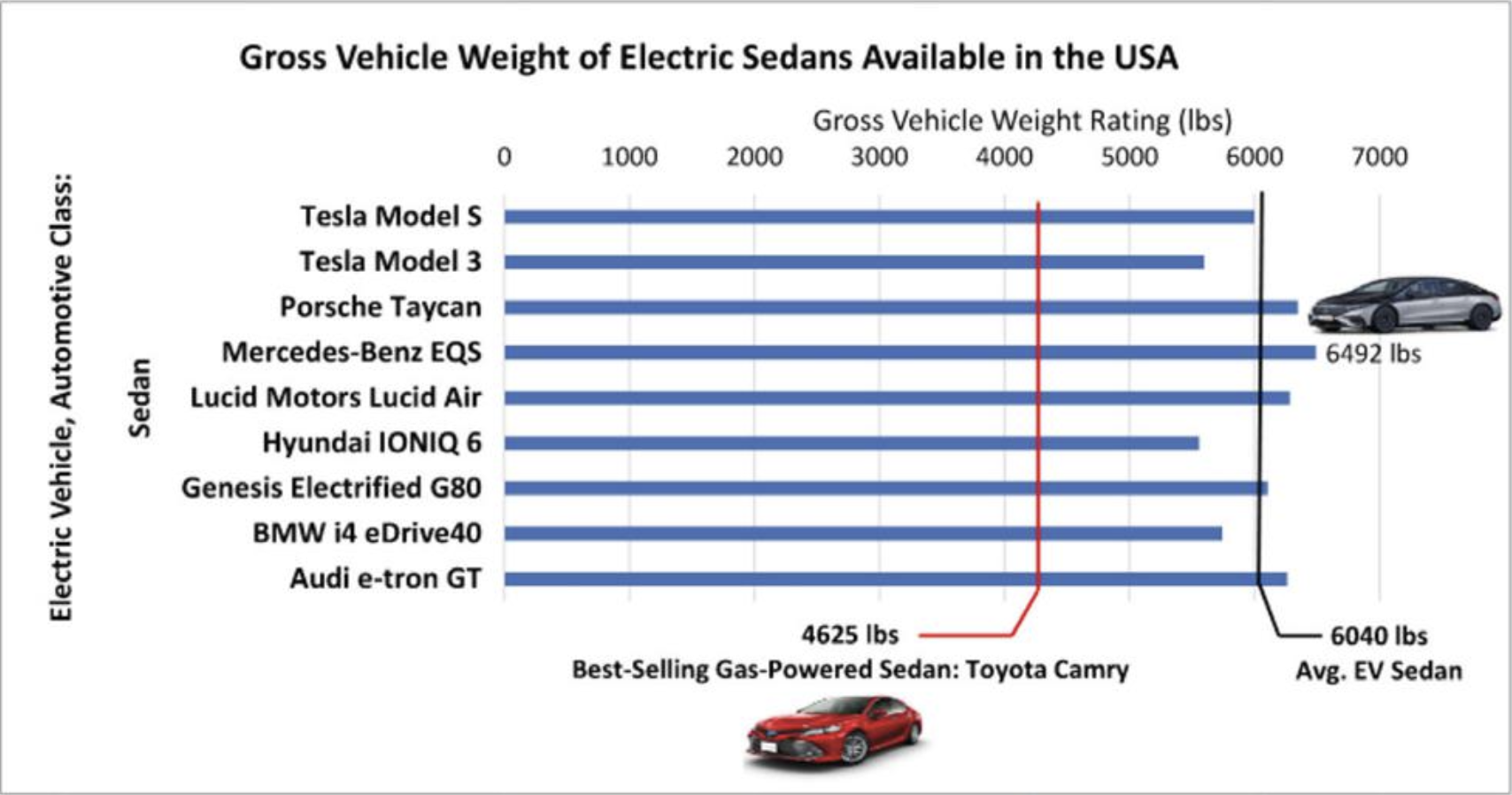
Although the increased weight of EVs may be a marginal issue if they only represent a small fraction of cars on the road, the percentage of EV cars in the U.S. has been on the rise, and sales estimates expect a dramatic increase as a part of the vehicle stock. In 2021, EVs only accounted for 3.2% of U.S. auto sales, but in the last two years, they have more than doubled and now represent 7.2% of U.S. auto sales in the first quarter of 2023 (Kelley Blue Book). In fact, as of Q1 2023, the Tesla Model Y has surpassed the Toyota Corolla as the best-selling car in the world. This rapid increase is caused by rising EV popularity and new U.S. legislation aimed at decreasing carbon emissions. According to industry analysis from HIS Markit, U.S. EV sales could reach 25 to 30% of total passenger car sales by 2035 and 45 to 50% of passenger car sales by 2050, under the assumption that the U.S. continues to tighten environmental legislation and at least five states complete the internal combustion engine ban by 2035 (HIS report).
Review of Historical Building Code Requirements
Modern EV weights are quickly approaching, and in some cases exceeding, the structural loads specified for design in current building codes. In contrast to the increasing vehicle weights and increasing numbers of EVs in the United States, the strength requirements for parking garage design have been reduced over the last two decades. In 2002, ASCE 7-02 reduced the minimum uniform distributed live load for passenger vehicle garage floors from 50 to 40 psf. At the same time, ACI 318-02 reduced the concrete design load factors for live load from 1.7 to 1.6. The product of these changes reduces the factored design live load by 25%, from 85 psf in 2002 to 64 psf today.
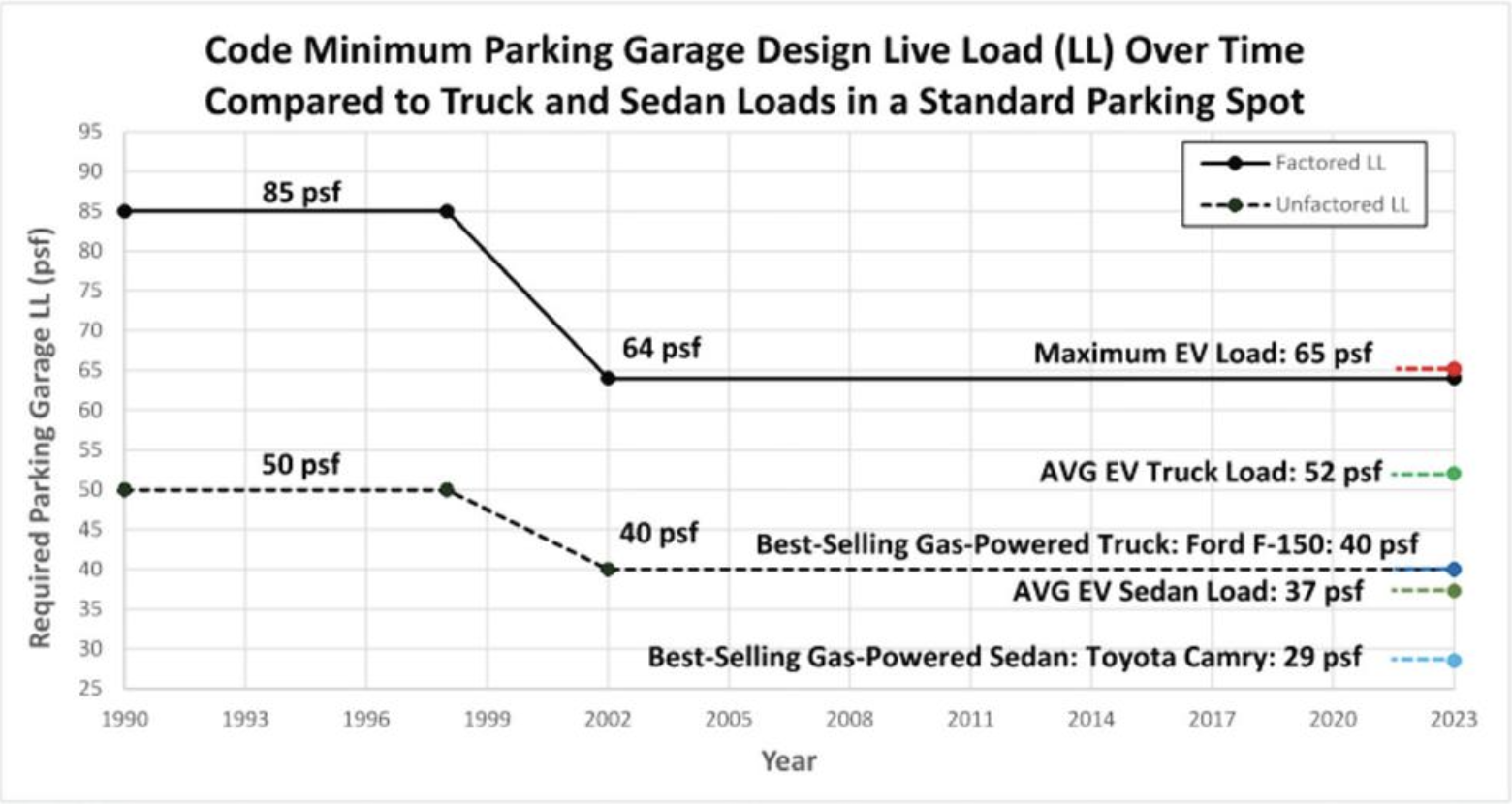
We converted the GVWR of the vehicles to area loads in a parking garage to compare the manufacturer’s data to the code requirements, as depicted in Figure 3. We used a standard parking space dimension of 9 feet by 18 feet as a simple representation of the area occupied per vehicle. Then, we compared uniform area loads of EV and internal combustion engine vehicles to the code-specified minimum design live load over time. The average unfactored load for an EV truck is 52 psf, 12 psf higher than the gas-powered Ford F-150, and the average EV sedan is 37 psf, 8 psf higher than the best-selling ICE sedan. When considering the current heaviest EV vehicle, the GMC Hummer EV pickup truck, the unfactored EV load in a 9 feet by 18 feet parking space could be as high as 65 psf.
The public trusts regulators, building officials, and engineers to keep the code conservative when considering live load over the building life. According to ASCE 7, the required live load used in the design of buildings and other structures shall be the maximum load expected by the intended use or occupancy. e Commentary of ASCE 7 further explains that the minimum uniformly distributed live loads provided in ASCE 7 are usually far higher than loads measured in live load surveys; however, the buildings must be designed for loads they are likely to be subjected to during some reference period, typically fifty years. Our research shows that the average parking space area loads of EVs are near to or greater than 40 psf, the current minimum live load required by code. Even the gas-powered Ford F-150 is right at the code-specified live load of 40 psf. While most EV vehicle loads are still less than the factored minimum live load, 64 psf, live load factors are meant to consider the variability of live load that could occur over time in the garage, and many parking garages in the United States are consistently filled with vehicles. With the popularity of EVs on the rise, building codes will need to revisit the live load requirements to keep up with increasing vehicle weights. e consequence of inaction is not a sudden wave of parking garage failures in the short term, but rather an increase in parking garage failures and maintenance costs over the long term.
Serviceability Considerations
The serviceability of parking structures includes various considerations that are beyond code minimum load requirements, such as concrete cracking, structure durability and corrosion resistance, wear on the driving surface, and structure vibrations. Neglecting or not considering many serviceability items will increase the owner’s maintenance costs and decrease the structure’s performance. The serviceability considerations listed above are subject to increased demands with the increased EV weights.
The American Association of State Highway Officials (AASHO) Road Test in the 1950s resulted in the Law of the Fourth Power, which postulates that increased axle weight increases road damage by the ratio of the increased weight to the fourth power. For example, a 30% increase in axle load is likely to increase repair costs by 185% (nearly tripled). A similar theorem could be applied to the supported decks of a parking garage. However, without the support of a road base, parking decks are likely to be even more vulnerable to deterioration due to increased axle loads.
There are a variety of typical parking garage construction types: reinforced concrete, topped and untopped precast prestressed concrete (precast double tees), post-tensioned concrete, or steel structures. They all have one element in common: the horizontal structure is concrete. Concrete is a great material to support vehicle loads in parking structures because of its strength, toughness, and durability when designed appropriately. However, all concrete is subject to cracking and can degrade the performance of the structure over time.
Reinforced concrete is the most ubiquitous horizontal framing material in parking structures and is subject to flexural demands from gravity loading. Flexural cracks in concrete form when the flexural stresses exceed the modulus of rupture of the concrete. To provide an economical structural design, beams and slabs are designed for strength with little consideration for limiting the tension stress in the concrete. Therefore, cracks are expected to form in locations with higher flexural demands, as shown in Figure 4.
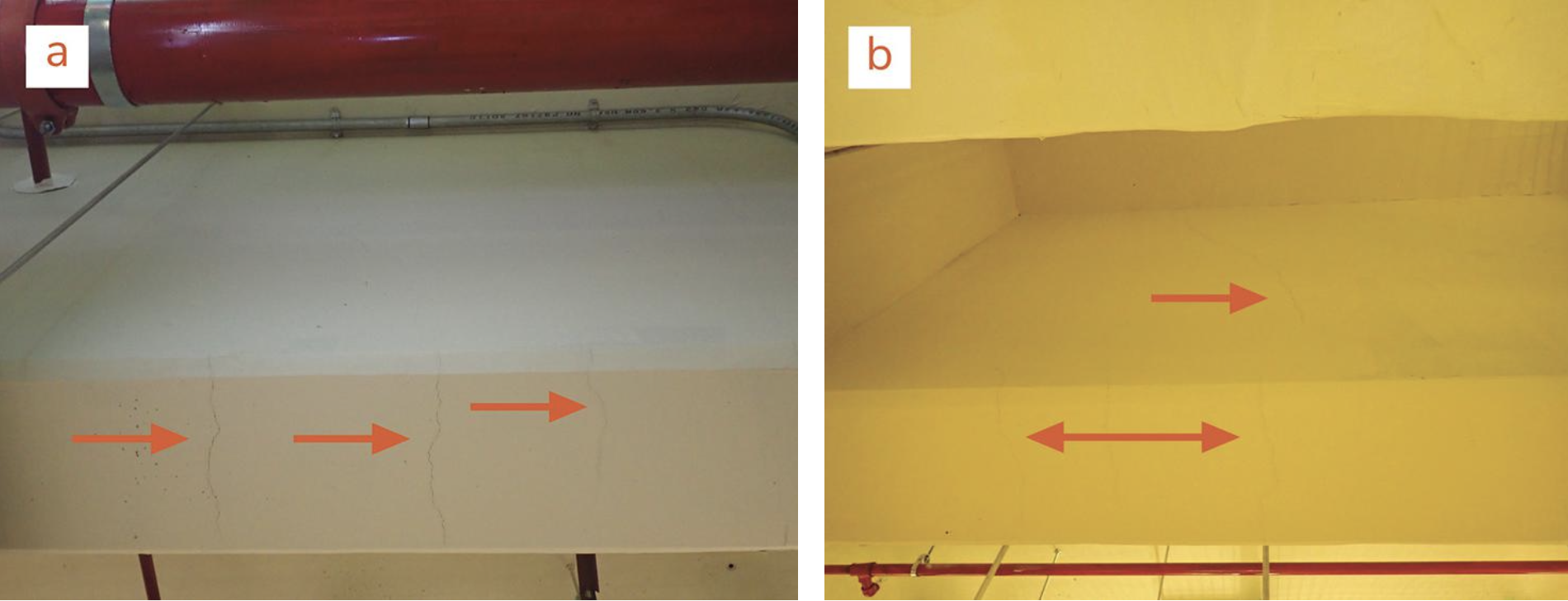
Cracks are the conduit for corrosive elements to penetrate concrete. Corrosive elements can include but are not limited to, chlorides from the environment, dirty water, carbon dioxide, and deicing salts. Chlorides are particularly corrosive to reinforcing steel, and the steel is typically located in the tension regions of concrete where cracking is concentrated. The increased loads from EVs increase the crack numbers, widths, and depths, which may decrease the life of a parking structure. EV charging stations are commonly grouped to make electrical distribution systems more cost-effective. Grouping EV charging stations will concentrate heavier vehicles, intensifying cracking.
A simple analysis can demonstrate how insidious increased loads can be for concrete beam and slab serviceability. For a simply supported beam or slab, the length over which the bending stress exceeds 90% of the maximum bending stress for a given uniform distributed load (i.e., imposed by ICE vehicles) increases by 73% when the uniform distributed load increases by 30% (additional load from EVs). In addition, the cracks will be wider and deeper. It is easy to understand why repair costs balloon with increasing loads.
Traffic coatings (waterproofing barriers) are commonly used to protect horizontal surfaces on parking structures. When properly maintained, a traffic coating system can significantly prolong the life of a parking structure. Traffic coatings are common on each level of parking structures in northern climates where deicing salts are common and tend to only be on the top exposed level in southern climates, if at all. The service life of traffic coatings can vary from 5 years for a lower-grade system to 20 years for a high-performance coating system. Heavier vehicles will increase wear on all traffic coatings due to increased friction and abrasion. Increased coating wear is common around corners, speed bumps, ramps, and braking zones.
Vibrations in parking structures can be a nuisance to walking occupants, who may perceive the structure as not performing properly. In the extreme case, the occupant can associate the vibrations with a structural load capacity concern. Vibrations in parking structures are commonly caused by vehicles moving through the structure and are more pronounced at movement joints, precast double-tee joints, and speed bumps. The vertical offset across each of these locations causes vehicles to impose a dynamic load on the structure. Vibrations created by electric vehicles are significantly greater than by ICE vehicles because of their weight, and the structure will be more susceptible to low-frequency excitation due to additional cracking.
To explore the potential structural impacts of higher EV weights in parking garages, the authors created and analyzed a computer model of a typical mildly reinforced concrete parking structure beam. e model beam span is 60 feet with a tributary width of 10 feet. The first step was to determine the code-level service demands on the beam by combining the effects of dead load and the code-standard 40 psf live load. Using this combination as our baseline, we subsequently replaced the 40 psf live load with some of the vehicle weights discussed above and compared the total service demands with the code case. As shown in Table 1, a parking garage supporting primarily EV sedans will very nearly exceed the code service loads, which are intended to be conservative. Heavier EVs will exceed the code service loads—some by significant margins.
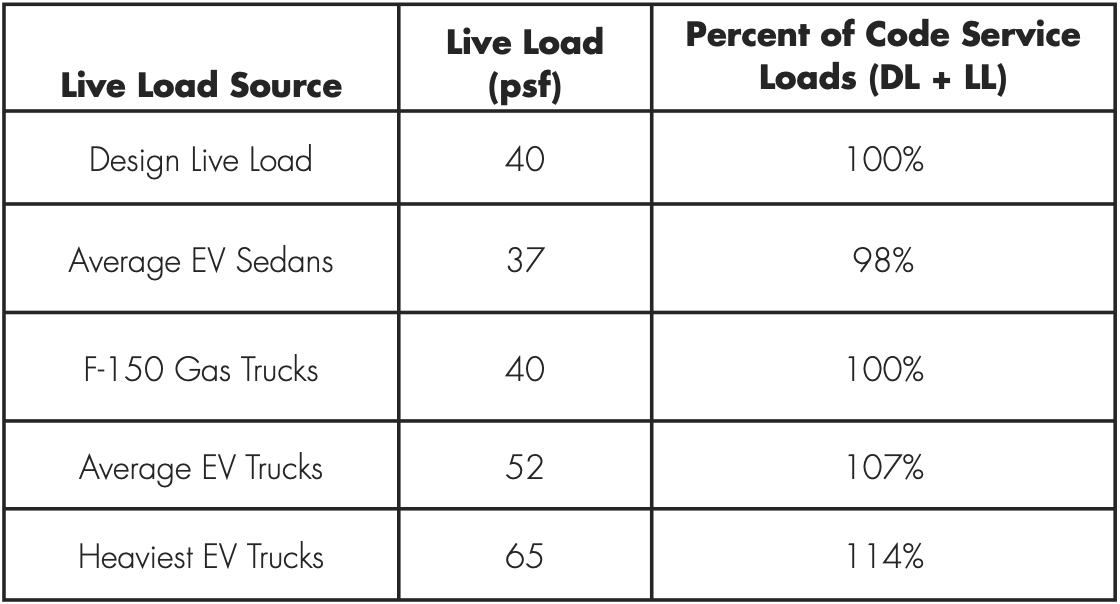
Vehicle Crash Barrier Public Safety
Vehicle crash barriers are a critical component of public safety in parking structures. The primary intent is to restrain moving vehicles and prevent out-of-control vehicles from crashing through. There are several recorded incidents where vehicle crash barriers have failed and resulted in fatalities. ASCE 7 species that vehicle crash barrier systems must withstand an impact force of 6,000 pounds at a height of 18 to 27 inches. Developing the required impact force is complicated and varies considerably based on the vehicle’s weight, speed, and energy absorption of the bumper system. Bhargava and Alostaz conducted an extensive study on vehicle impact forces (Bhargava & Alostaz, 2019). e study calculated impact forces by using high-fidelity, physics-based, three-dimensional finite element simulations of various vehicles crashing into vehicle barriers. e study concluded that the smallest vehicle (2,938 pounds) traveling at 5 miles per hour exerted an impact force of 8,990 pounds on a vehicle barrier. The study also demonstrates that the impact force is not linear between the various vehicle weights and that it increases with increased crash barrier stiffness. All the scenarios in the study concluded that the code-required impact force is inadequate. Public safety is decreasing with respect to vehicle crash barriers in parking garages due to the greater EV weights.
Consumer Demands
In addition, the single biggest performance constraint that limits EV marketability is range. Increased battery size or technological breakthroughs with greater battery density are potential sources of significantly increased range. It is a prima facia truth that designers will not constrain increased range based on vehicle weight alone. Hence, the EV range challenge has significant potential to exacerbate the infrastructure challenge.
Conclusion
EVs weigh significantly more than their ICE counterparts on which the structural codes were based, and shortly before the popular advent of EVs, the code design requirements were reduced. The disparity between growing loads and reduced design requirements is reducing the margin of safety, increasing the likelihood of structural failure, and will exponentially increase maintenance costs for code-compliant parking structures. For non-compliant parking structures, the consequences could be even more severe. Consequentially, it is possible that the risk of catastrophic structural failures in the future could jeopardize the viability of EV technology as part of national efforts to reduce carbon emissions. Engineers and contractors have an opportunity to contribute to providing sound infrastructure to enable EV technology to be part of the fight against climate change.
The authors recommend that engineers and code officials, in collaboration with specialty contractors, implement the following actions with respect to EVs to address this predicament:
- Review code load requirements and implement changes as needed.
- Survey authorities having jurisdiction and industry organizations (such as AASHTO) for best practices for mitigation.
- Perform detailed structural analysis of common parking garage construction types for increased loads.
- Develop standardized analysis and strengthening measures to make it more efficient and effective for owners to strengthen their parking garages.
- Publish detailed example analysis and strengthening projects as models for assessment and upgrade.
- Execute a program of monitoring, measuring, and documenting parking garage performance with significant EV occupancy.
- Consider limiting EV access, and especially charging stations, to parts of parking garages less vulnerable to load effects, such as slab-on-grade areas or limited portions of the parking garage strengthened for greater live loads.
- Consider vehicle fleet weight cap equivalent to vehicle mileage cap.
- Consider standard practice of distributing EV parking spaces to reduce load intensity.
References
ASCE, (2022). Minimum Design Loads for Buildings and Other Structures. Reston: American Society of Civil Engineers.
Bhargava, A., and Alostaz, Y. (2019). Estimation of Vehicular Collision Force for Barriers. Structures Congress 2019.
ICC, (2020). 2021 International Building Code. International Code Council.