Advancing First-Generation Performance-Based Seismic Design for Steel Buildings
Part 3: Future Efforts for All Structure Types
Capabilities to conduct a performance-based seismic design (PBSD) of retrofitted existing buildings and new buildings have advanced exponentially over the past 25 years. This progress has augmented our knowledge of building behavior given an earthquake intensity. Still, we must be cautious of considering a PBSD as an exact answer; instead, a PBSD gives us information to support decision-making. There is still much work needed to support PBSD capabilities, and this depends on the type of assessment being conducted. At the same time, a vision for the not-so-distant future must also be established.
The previous two parts of this series on advancing first-generation PBSD principles and provisions for steel buildings (STRUCTURE, October and November 2021) discussed the history of PBSD. They also outlined a project initiated at the National Institute of Standards and Technology (NIST) that evaluated what advancements could be made. That project started by benchmarking ASCE 41 to ASCE 7 to develop a baseline.
This third and final article highlights several concepts that could advance current PBSD capabilities. This article goes beyond steel buildings and takes a heuristic view of needs for all types of building construction, non-building structures, and lifeline infrastructure (generically referred to here as a system). These concepts can apply to both first- and next-generation PBSD and include the following:
- intrinsic risk assessment;
- procedures and metrics to evaluate functional recovery time;
- multi-system coordination; and
- resilience-based seismic design.
These concepts may be initiated by NIST or by any partner agency in the National Earthquake Hazards Reduction Program (NEHRP). Realization of these concepts will be dependent upon available resources.
Intrinsically Evaluating Risk Exposure
First-generation PBSD principles contained in the latest edition of ASCE 41: Seismic Evaluation and Retrofit of Existing Buildings (ASCE 2017) fundamentally result in a component-level binary pass or fail evaluation. A consequence of this process is that component performance and the potential need to retrofit or replace is based upon an analysis output rather than the effect that the component performance has on the system’s overall performance. Therefore, an engineer cannot effectively use the assessment results to explicitly evaluate risk, which is highly dependent upon the degree of redundancy built into the building.
As an example, assume that an assessment is being conducted for the collapse prevention (CP) structural performance level (SPL) at the risk-targeted maximum considered earthquake (MCER) prescribed in ASCE 7, Minimum Design Loads for Buildings and Other Structures (2010 edition and later). Furthermore, suppose the collapse risk is taken according to ASCE 7. In this case, the question becomes what percentage of components needs to fail the CP SPL to achieve a 10 percent probability of collapse given MCER shaking? Since there is no mechanism to assess risk based on the analysis results, exposure to risk cannot be communicated to shareholders and stakeholders.
A mechanism is needed to relate failure (for any performance level) of components based on consequences posed to the building owner, occupants, service users, etc. An example of such an approach could be that a building poses more than a 10% probability of not satisfying a performance target (based on collapse, economics, loss of function, etc.) if either of the following occurs:
- more than some percentage of the total structural components in one direction do not satisfy the target performance level; and
- more than some percentage of the structural components resisting seismic force or deformation in one story in one direction do not satisfy a target performance level.
The challenge would be defining the percentages in a codifiable manner for policymakers and easily understood by the public. NIST GCR 12-917-20: Tentative Framework for Development of Advanced Seismic Design Criteria for New Buildings (NIST 2012) started evaluating risk targets for new buildings for adoption by ASCE 7. The same process can advance ASCE 41 using the methodology given in the Federal Emergency Management Agency’s (FEMA) P-58: Seismic Performance Assessment of Buildings (FEMA 2015), which can explicitly evaluate seismic risk in a probabilistic sense.
Functional Recovery Time
A common consequence of an earthquake is interruption of building functions and operations and community support services (e.g., power or water distribution). These downtimes can range from a few hours to years. Recovery time for a building is impacted by the following, to list a few factors:
- the extent of damage to building systems (structural or non-structural) and the operative contents used for services;
- availability of financial and damage assessment resources;
- demolition and repair processes, including mobilization time;
- construction material availability; and
- recovery of lifelines supporting building function and operation.
Recovery time may also be tied to community-level indirect losses such as loss of employment, displacement, and interruptions to education, childcare, and community services. This is discussed later in this article. Anecdotally, after the M7.1 Anchorage, AK, earthquake in November 2018, a building housing a notable coffee service sustained ceiling damage (non-structural). The business continued the next day by removing the ceiling to avoid such losses.
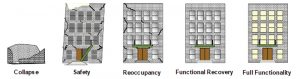
There is a need to develop and implement enhanced performance levels and PBSD guidance that address post-earthquake re-occupancy and functional recovery time. Figure 1-a shows an example of what the performance continuum may look like within this performance objective. Comparing Figure 1-a to Figure 1-b (also included in Part 1 of this series, October 2021) indicates that there may be cases when functional recovery governs performance and other cases when collapse prevention governs, but in no instance shall collapse prevention be overlooked. Within this effort, a set of distinguishable terminologies must be developed as well as a clear understanding of the result. For example, function and operation may be interpreted differently within the same organization. Is continuity of operations by providing services elsewhere (or in their parking lot) deemed to satisfy recovery requirements? Does the case satisfy re-occupancy requirements when the only elevator in a building is down, and one stair system is operational when the building may serve users who cannot use stairs? These are just a few of the multitude of inquires that need to be addressed by architects and engineers to define the needed metrics. Additional topics can be found in NIST SP 1269: NIST-FEMA Post-Earthquake Functional Recovery Workshop Report (NIST 2021b), which summarizes the feedback received by workshop participants on functional recovery concepts and options.
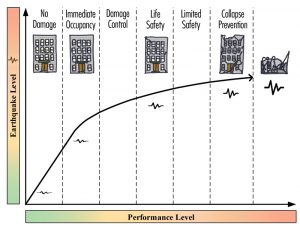
In the latest reauthorization of NEHRP (Public Law 115-307, December 2018), NIST and FEMA were tasked to report on recommendations for improving the built environment and critical infrastructure to reflect performance goals stated in terms of post-earthquake re-occupancy and functional recovery time. Their report, FEMA P-2090 / NIST SP-1254: Recommended Options for Improving the Built Environment for Post-Earthquake Reoccupancy and Functional Recovery Time (NIST 2021a), identified seven recommendations for design or retrofit of buildings and lifeline infrastructure that would culminate in a framework to address re-occupancy and functional recovery time.
Multi-System Coordination
The results from a current PBSD, either using ASCE 41 or FEMA P-58, tend to focus on assessing the design or retrofit of a single building. This approach caters to a single isolated building or even an organization where operations within multiple buildings are mutually exclusive. However, it does not address interactions among multiple associated buildings, among multiple lifeline infrastructure sectors, or between a combination of the two. For example, how does the performance of one school across town affect another school with regards to consequences to the school district? Assume that a school is closed due to earthquake damage (Figure 2). In this example, the students are required to go to another school; however, that school is too small to handle the increased student population. Consequently, the school splits classes and holds some on Saturdays. Further, how does the school district coordinate with the local Department of Transportation to address adjusting school operations based on changes in traffic pattern demands?
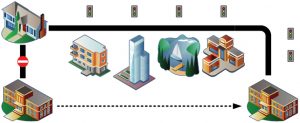
There is a need to enhance PBSD to support integration across systems and sectors beyond prioritization by risk categories. For example, allowing multiple systems to provide feedback to other potentially impacted systems during the design or assessment process enhances risk assessments and associated decision metrics.
Resilience-Based Seismic Design
The conceptual difference between PBSD and resilience-based seismic design (RBSD) is that the latter evaluates the performance of a system with regards to its impact on the performance of a more extensive network of building and lifeline infrastructure systems and sectors. RBSD is thus a potential mechanism to evaluate impacts on community resilience. With that said, there may be conditions when the performance of a system does not impact community resilience, and the prioritization of network systems must be made at the community level. For example, should a building housing a large construction material retailer be designed as an ordinary building if downtime can hinder the recovery progress of the residential market it serves?
PBSD can be used to estimate whether a design is highly likely to be functional after an earthquake (e.g., sustains less damage). In so doing, the system will contribute to the community’s resilience. PBSD can be augmented to include the impact of utilities and services needed for the system to regain function and operations, but it is still focused on a system. RBSD can employ PBSD and incorporate prioritized community resilience concepts such as addressing impacts to the transportation network and, in turn, how that system may impact other systems and services. Essentially, RBSD can be envisioned as a series of nodal enhanced PBSDs within a network communicating with each other.
RBSD must also address compounding consequences from coincidental hazards and/or sequential hazards and the societal responses to them. In this context, coincidental hazards are one or more hazards unrelated to the earthquake hazard that may occur within the same response and recovery period. Sequential hazards are one or more secondary hazards that directly result from the earthquake that may occur within the same response and recovery period.
A metric for earthquake resilience is challenging to define, beyond qualitative characteristics – having the ability to withstand, respond to, and recover from an earthquake and its consequences, and not just one earthquake. Moreover, quantitative assessment of resilience can only be measured after the impact on a network of systems from an earthquake is known because response and recovery are time-dependent functions without pre-defined timelines. Therefore, the subsequent resilience score (for the next earthquake) is a function of the measurable change in resilience based on mitigation efforts, repair or improvements, availability of construction resources, and economic impacts, to list a few. However, an aspect of resilience that is more challenging to quantify is its impact on society.
The engineering community must be able to communicate risk exposure to shareholders and stakeholders. The performance of a building must be able to address the welfare of its occupants or the public that use the services provided to progress. It is straightforward with PBSD to address physical damage and downtime of the physical structure as a primary indicator of performance. The losses from consequences on society such as mental and physiological health, displacement, interruptions to education, work, childcare, and community services play a key factor in estimating the holistic performance needed in RBSD.
Conclusion
This article discussed several future concepts to advance PBSD. These concepts can be somewhat aspirational but nonetheless outline the needs for progress that, when integrated, build upon each other. PBSD is not a tool strictly used to circumvent prescriptive building code provisions or save upfront construction costs, though this has been a result. Instead, PBSD provides a rational estimate of design performance in a future earthquake. It must also be used to understand the associated risks that such a design may pose to the community it serves. Unfortunately, decision-makers generally only see part of the picture. Absent appropriate financial incentives, public and private organizations tend to invest in measures that they believe protect their economic welfare, not necessarily those that augment the community’s wellbeing.
With the current trend towards defining and implementing resilience measures and guidance, it is difficult to continue along the path where new and existing buildings can be treated differently. In the eyes of the public, there is no difference in the function and operations of either. It could be possible within the context of RBSD to envision ASCE 41 and ASCE 7 as one standard. Seismic safety is a choice based on risk, and the developed tools need to address these risks so that users may augment them as needed and set priorities to benefit the community they serve.■
References
FEMA (2015). Seismic Performance Assessment of Buildings – FEMA P-58. Federal Emergency Management Agency. Washington, D.C.
NIST (2012). Tentative Framework for Development of Advanced Seismic Design Criteria for New Buildings – NIST GCR 12-917-20. Produced by the NEHRP Consultants Joint Venture, a partnership of the Applied Technology Council and the Consortium of Universities for Research in Earthquake Engineering, for the National Institute of Standards and Technology, Gaithersburg, MD. www.nehrp.gov/pdf/nistgcr12-917-20.pdf. Last verified 10/01/2021.
NIST (2021a). Recommended Options for Improving the Built Environment for Post-Earthquake Reoccupancy and Functional Recovery Time – FEMA P-2090 / NIST SP-1254. National Institute of Standards and Technology, Gaithersburg, MD and Federal Emergency Management Agency, Washington, D.C. https://doi.org/10.6028/NIST.SP.1254. Last verified 10/01/2021.
NIST (2021b). NIST-FEMA Post-Earthquake Functional Recovery Workshop Report – NIST SP 1269. National Institute of Standards and Technology, Gaithersburg, MD. https://doi.org/10.6028/NIST.SP.1269. Last verified 10/01/2021.