This is the second of a two-part series on the Kahramanmaraş Earthquake Sequence which occurred on February 6, 2023 in Turkiye. The first article was printed in the STRUCTURE magazine July 2023 issue and presented a background on regional seismicity, seismic design and practice in Turkiye, and the authors’ observations from the field. This article focuses on observations relevant to structural practice in the U.S.
Relevance of the Kahramanmaraş Earthquake Sequence to the United States
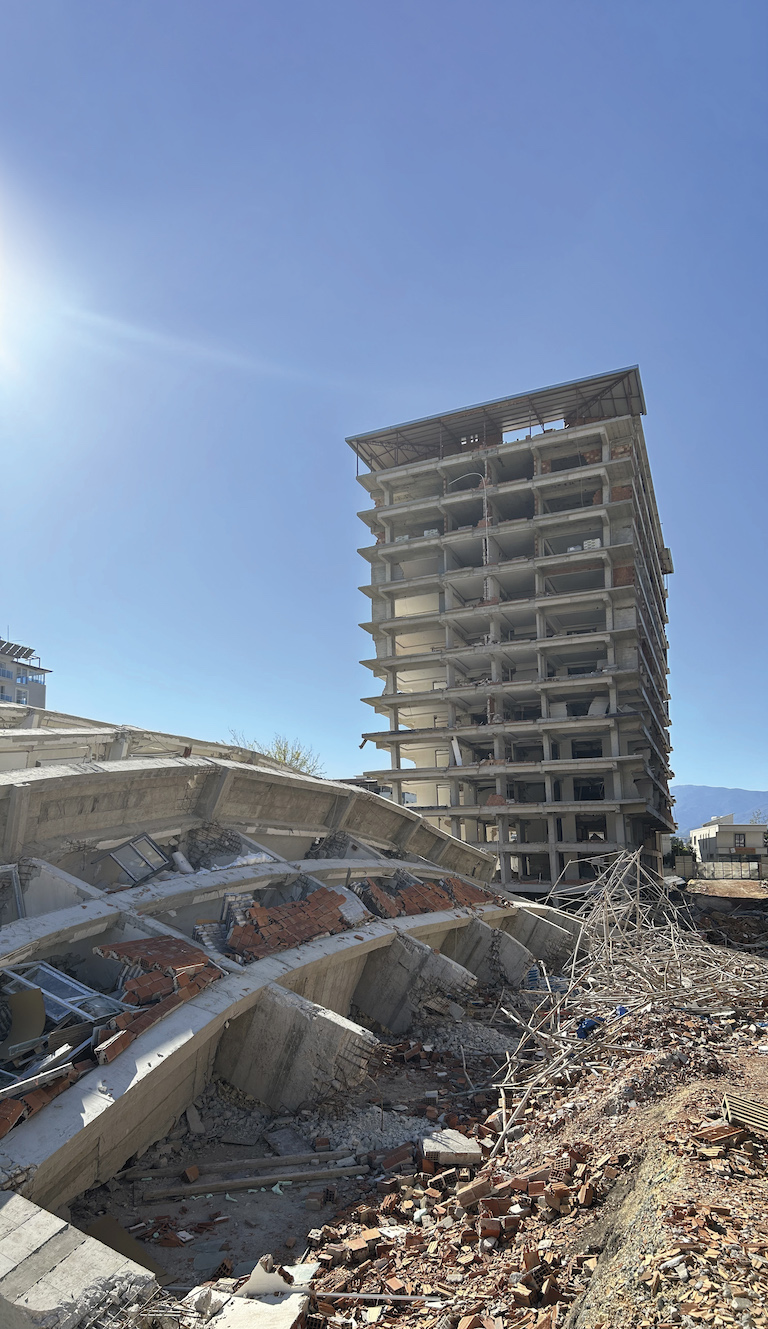
Given the spatial separation and cultural differences between Turkiye and the United States, it is easy to fall into the mental trap of preemptively concluding that the damage and casualties seen in the Kahramanmaraş Earthquake Sequence are not representative of what may occur in the United States. And while it is true that differences in design, materials, construction, inspection, and enforcement do exist, it is important to consider that:
The building stock in the U.S., similar to Turkiye, is a mix of older and newer buildings in proportions that reflect time periods of growth for different cities. For example, nonductile concrete buildings constructed before the 1980s in western U.S. cities exhibit at least as poor detailing and strength characteristics as those of more modern reinforced concrete buildings in Turkiye, many of the latter of which were severely damaged or collapsed.
Even for new Risk Category II buildings in the U.S., a 10% probability of collapse given the Risk-Targeted Maximum Considered Earthquake (MCER) is all that is assured in ASCE 7. While many engineers have and continue to argue that this is a conservative estimate of collapse for what actual designs produce, it is still a pressing reminder that the building code in the U.S. targets reducing the probability of collapse, not eliminating it. For a brief overview of collapse targets in ASCE 7, please see “Acceptable Collapse?” in the March 2015 issue of STRUCTURE magazine (https://www.structuremag.org/?p=8164).
Seismic shaking intensities over the geographic and population extent subjected to the Kahramanmaraş Earthquake Sequence in Turkiye have never been experienced in the U.S. to date. Notably, the shaking intensities experienced in the Kahramanmaraş Earthquake Sequence exceeded the average values computed by ground motion prediction equations, the same or similar ground motion prediction equations which are used to derive seismic hazard demands in U.S. building codes.
With a better understanding of the devastating effects of the Kahramanmaraş Earthquake Sequence, structural engineers in the U.S. may begin to question the futility of attempting to change the outcome of a similar earthquake in the U.S. However, the authors encourage U.S. practitioners to instead be reminded of structural engineers’ essential role and the immensely meaningful impact we can make. The following sections discuss observations from the Kahramanmaraş Earthquake Sequence which are especially relevant to U.S. practice.
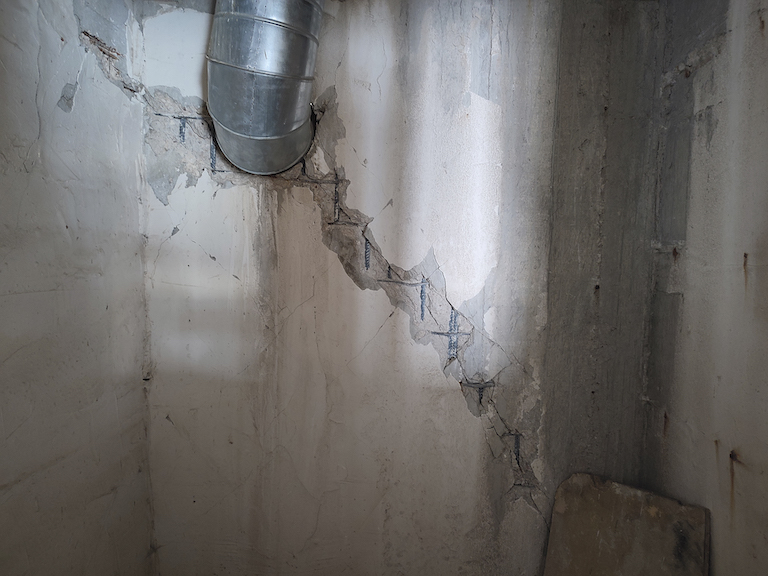
Structural Observation and Special Inspection are High-Value
Structural observation and special inspection are extremely high-value tasks, meaning they produce large benefits to building performance for relatively lower levels of effort/time. Structural observation and special inspection are often code-required in the U.S., particularly in the higher seismic regions of the western states. This requirement is sometimes seen as a burden by structural engineers to make time for a site visit in an already too-busy schedule. Due to contracting practice in Turkiye, it is very uncommon that the structural engineer who prepares the contract documents also has the opportunity to observe or inspect their design during construction. Even for significant structures, the authors are aware of instances in Turkiye where the structural engineer who prepared the drawings had their first opportunity to visit the building only after the earthquake. In Turkiye, a separate field engineer is hired who often has no prior relationship to the design. The authors observed multiple buildings for which construction differed from the prescribed design (e.g., missing or inappropriately spaced rebar stirrups and ties in reinforced concrete columns, conduit or duct interrupting structural elements, etc.) (Figure 1). These seemingly small details, which the design engineer understands fundamentally and would recognize immediately if on site, can have major detrimental impacts to building performance. Therefore, the authors encourage U.S. practitioners to remember that structural observation and special inspection are much more an opportunity to enhance public safety than a burden on their time.
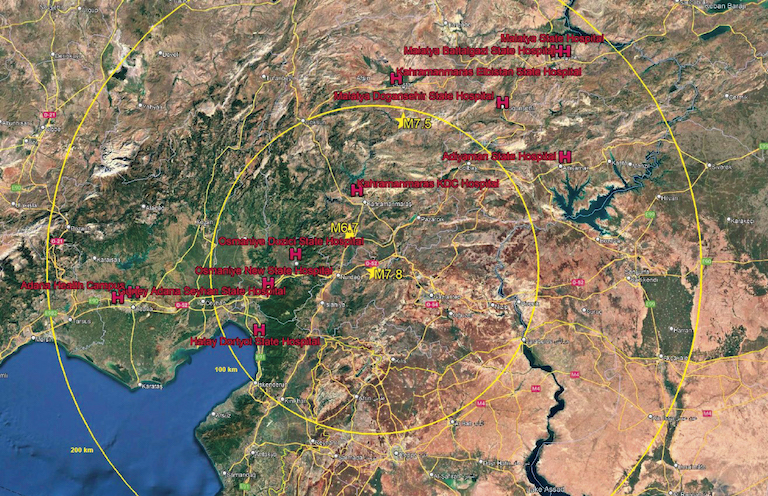
Seismic Isolation Works
Seismic (base) isolation works and, if not the only appropriate solution, it is at least by far the best option for certain occupancies such as acute-care hospitals in a high seismic region. The Ministry of Health for Turkiye released a regulation in 2013 requiring that all new hospitals with a bed capacity greater than 100 be constructed using seismic isolation. Of the approximately 100 seismically isolated buildings in Turkiye, eleven seismically isolated hospitals were within the affected region of the Kahramanmaraş Earthquake Sequence (Figure 2). All performed very well, particularly in comparison to the significant structural damage or collapse exhibited by fixed-base buildings. Although the first seismically isolated hospital in the U.S. (University of Southern California University Hospital which opened in 1992, also the first known modern seismically isolated hospital in the world) was constructed almost a decade prior to the first in Turkiye (Kocaeli University Hospital in 2001), Turkiye has now far surpassed the U.S. in the total hospital floor area protected by seismic isolation as a result of the Ministry of Health regulation. Some of the reasons for the stagnation of seismically isolated buildings in the U.S. are described in “What’s Happened to Seismic Isolation of Buildings in the U.S.?” in the March 2012 issue of STRUCTURE magazine (https://www.structuremag.org/?p=4166). While the performance of seismically isolated buildings in Turkiye was not perfect, owing primarily to issues with structural observation and inspection of moat clearance, the Kahramanmaraş Earthquake Sequence may be a wake-up call for increased seismic isolation of acute-care facilities in high seismic regions of the U.S.
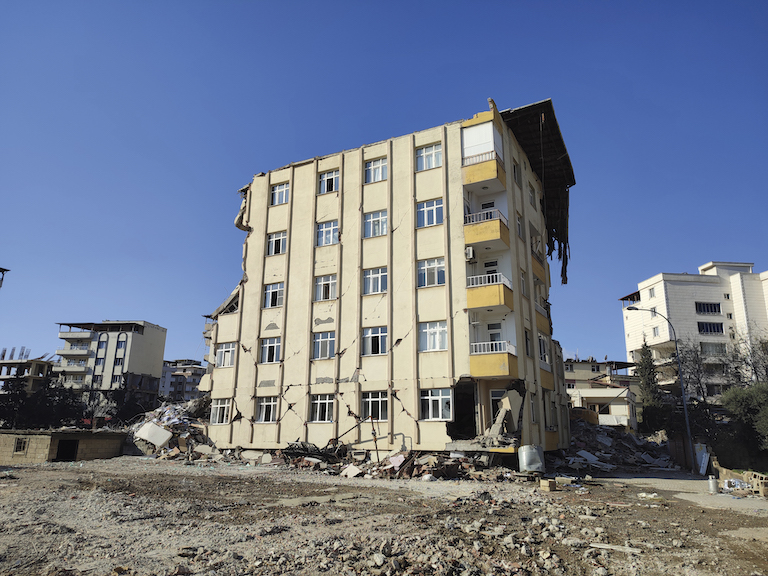
Seismic Retrofitting Occurs Slowly
Seismic retrofitting of existing buildings in the U.S., in combination with demolition and rebuilding, is a necessary component of improving life-safety on a community level. The Turkish government enacted a tax following the 1999 Duzce and Golcuk earthquakes which was intended to fund seismic safety improvements. However, those funds did not appear to primarily be used for seismic retrofitting of existing buildings but were rather diverted to new infrastructure and large public works projects. An urban transformation law was also enacted with the aim of encouraging owners to retrofit or demolish and rebuild their older buildings through financial support. However, this funding did not appear to motivate a large percentage of existing building owners. As a result, the authors did not observe any seismically retrofitted buildings in the earthquake-affected zone of Turkiye. In the U.S., seismic retrofit occurs somewhat infrequently, typically either as a result of voluntary efforts by concerned owners or as mandated by jurisdictional rules or building codes. For example, the International Existing Building Code (IEBC) requires seismic evaluation and retrofit for a change of Risk Category or a significant alteration. Under the IEBC, alterations only trigger an evaluation and retrofit if more than a certain percentage of the gravity members are affected or if the lateral force-resisting system is significantly altered. Furthermore, certain local jurisdictions impose mandatory seismic retrofit when overall renovation costs per square foot exceed a certain limit. Such alterations are uncommon or, alternatively, the alteration is modified to avoid triggering an evaluation and retrofit. Additionally, multiple jurisdictions in the western U.S. have enacted ordinances for the seismic evaluation and retrofit of certain existing building types (e.g., unreinforced masonry, wood soft-story, non-ductile concrete, etc.). See Figure 3 for a weak/soft story collapse in Turkiye. Such mandates appear to be appropriately targeting the most seismically vulnerable existing buildings and yet the percentage of retrofitted existing buildings in the high seismic regions of the U.S. remains low. Furthermore, similar to Turkiye, seismic evaluation and retrofit mandates in the U.S. are susceptible to public and political pressures (e.g., competing demands with the housing crisis).
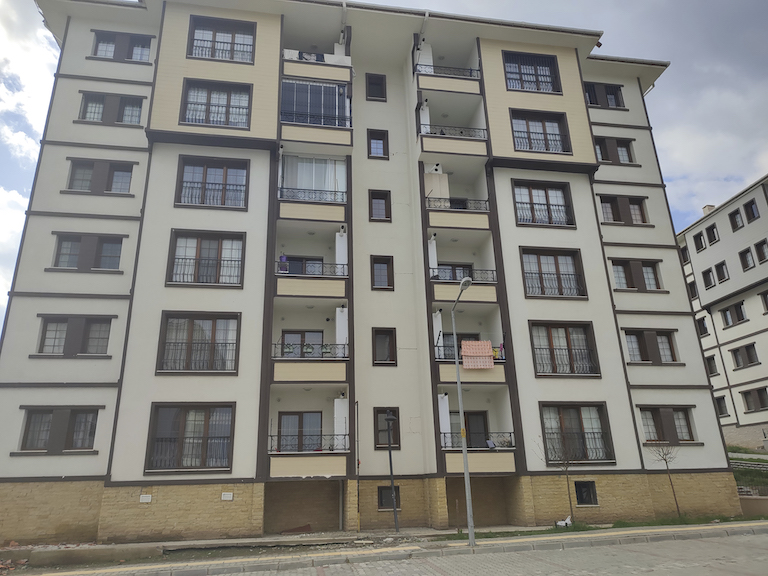
Functional Recovery for Existing Buildings May Detract from Life Safety
A growing focus in the U.S. is the shift to functional recovery design or, in other words, design for enhanced performance beyond life safety/collapse considerations and towards reducing building downtime. This practice is generally looked upon favorably as it could better align future U.S. codes with the public’s performance expectations for buildings. Furthermore, in new building design, some version of enhanced functional recovery can often be delivered through reasonable incremental construction costs. See Figure 4 for a tunnel-form building visited by the authors with negligible recovery time which was in a region of otherwise severely damaged buildings. Refer to Part 1 of this article published in the July 2023 issue for a description of tunnel-form construction. The idea of design for functional recovery is also beginning to be considered for existing buildings in the U.S. with the understanding that incremental construction costs are likely not as favorable as for new buildings. While this is commendable, if the Kahramanmaras Earthquake Sequence is any indication of even just older building performance in a similar earthquake in the U.S., it is clear that a safety-based performance objective still requires significant attention for existing buildings. This conclusion, therefore, begs the question as to whether functional recovery design for existing buildings in the U.S. is appropriate, especially when the larger incremental construction costs for functional recovery retrofit of existing buildings could instead be allocated to improving safety-based objectives (i.e., life safety seismic retrofit).
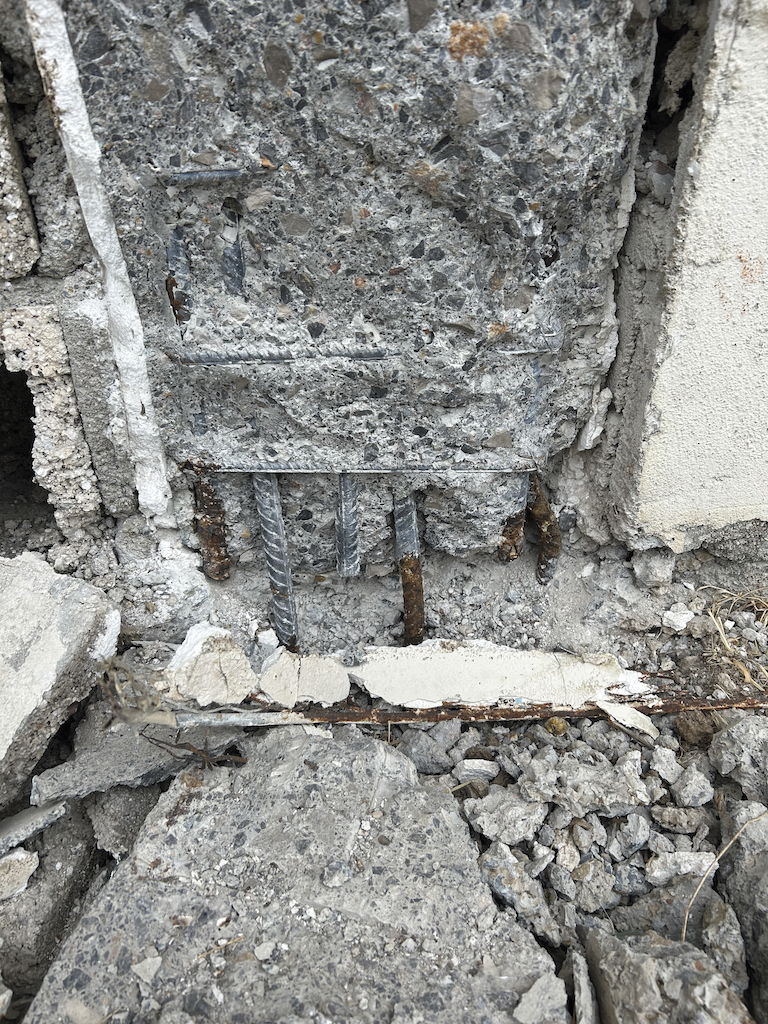
Reduced Drift Limits Can Help Compensate for Other Factors
Similar to U.S. building codes, the 2007 Turkish Building Earthquake Code permitted typical buildings to displace to as much as 2% story drift ratio in a design-basis earthquake. While both Turkish and U.S. engineers have the knowledge and capability to design structural systems to be stable and safe at those displacements, this quickly becomes complicated by errors in design or construction and nonstructural material availability and practices. For example, in multiple damaged buildings observed by the authors in Turkiye, column rebar dowels from the foundations were misplaced during construction and were bent over slightly to fit within the column core (Figure 5). This led to premature buckling and fracture of those bars (i.e., lesser ductility capacity than anticipated) in many instances and may have contributed to partial or total building collapse. As another example, nonstructural walls in Turkiye are almost exclusively constructed of unreinforced masonry due to material availability and labor knowledge. When these masonry walls are in-filled within a reinforced concrete frame, they are readily damaged at low levels of drift demand and also influence the response of the reinforced concrete frame (e.g., asymmetrical masonry damage in plan causing torsion, greater masonry damage at the lower stories causing weak/soft story formation, etc.). While both errors in design/construction as well as brittle nonstructural components can be addressed through other means (e.g., enhanced inspection and detailing, respectively), they can also be addressed by reducing allowable drift limits. For example, low-rise tunnel-form construction in Turkiye was observed to perform well (Figure 4) with high shear wall densities resulting in low drifts. Limiting drift, therefore, serves as a straightforward way of compensating for a milieu of other factors which would otherwise require interventions at multiple points in the design and construction process.
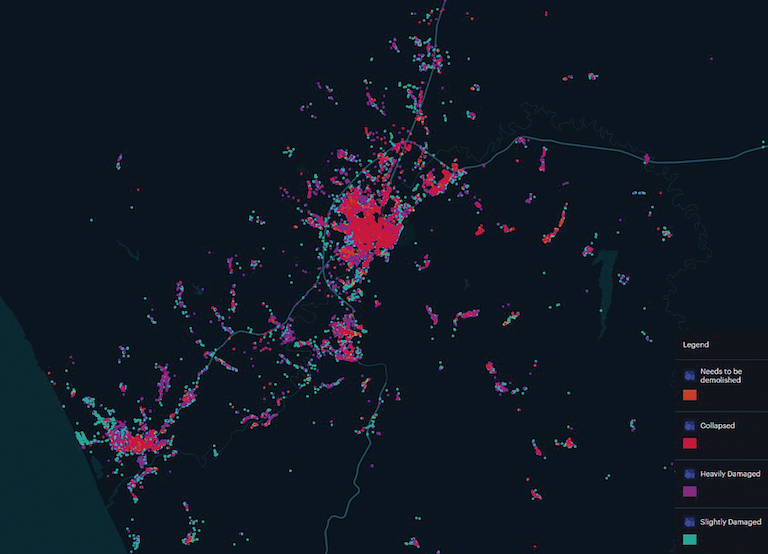
Post-Earthquake Inspection and Tagging Requires Communication
The authors regularly encountered residents in the earthquake-affected region of Turkiye who were unaware of the official safety and access evaluation for their building. Following the Kahramanmaraş Earthquake Sequence, an extensive evaluation process occurred to designate buildings as either Collapsed, Needs to be Demolished, Heavily Damaged, Slightly Damaged, or Undamaged using a rapid assessment involving a few minutes of observation by academics or government engineers (Figure 6). Despite this process, few to no physical tags were present and it was unclear whether residents were informed that the assessments were available online. In the U.S., post-earthquake tagging typically follows ATC-20 Procedures for Postearthquake Safety Evaluation of Buildings which involves placarding as Unsafe, Restricted Use, or Inspected after a rapid and, if necessary, a later detailed assessment. Yet, similar to cities in Turkiye, jurisdictions in the U.S. are likely to be ill-prepared to respond quickly to an event of similar intensity and extent to the Kahramanmaraş Earthquake Sequence due to lack of trained staff or lack of quick access to as-built drawings. Furthermore, while a rapid ATC-20 evaluation may only take tens of minutes, helping residents understand that evaluation and overcome the fear of entering a building that is even only cosmetically damaged is likely to take far longer.
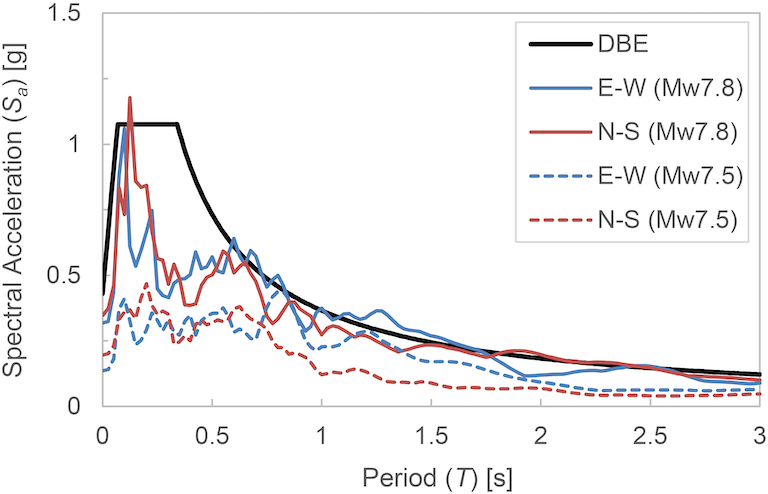
Earthquakes Consist of Multiple Events
Similar to the 2010-2011 Canterbury Earthquake Sequence in New Zealand where multiple high-intensity earthquakes occurred in series, the Kahramanmaraş Earthquake Sequence in Turkiye illustrated how earthquakes one after another can increase damage to buildings and loss of life. For certain buildings/sites, the Kahramanmaraş Earthquake Sequence produced near design-basis earthquake shaking compared to the Turkish Building Earthquake Code in the first event followed bysignificant, although not equal, shaking in the second event (Figure 7). As occurred in the Kahramanmaraş Earthquake Sequence, triggering of adjacent faults is also possible in the U.S. (e.g., multiple ruptures on the San Andreas Fault System). The authors visited a collapsed building in the city of Kahramanmaraş which had sustained damage but allowed everyone to evacuate during the first event (Figure 8). Several residents had re-entered to search the building and collect belongings when the second major earthquake occurred nine hours later. The building collapsed killing all those still inside. In the U.S., similar to Turkiye, the building code does not consider an explicit performance objective for multiple earthquakes in series. While the implied ability of a building to sustain aftershocks is often made, the quantity and intensity of those aftershocks and the explicit capacity of a damaged building to resist them are not quantified. This leads to questions about whether a building that survived a design-basis earthquake shaking and then collapsed in a high-intensity aftershock would meet the performance expectations of the U.S. building code. If aftershock resistance is expected of code-conforming buildings, explicit consideration and checks would need to be introduced into ASCE 7.
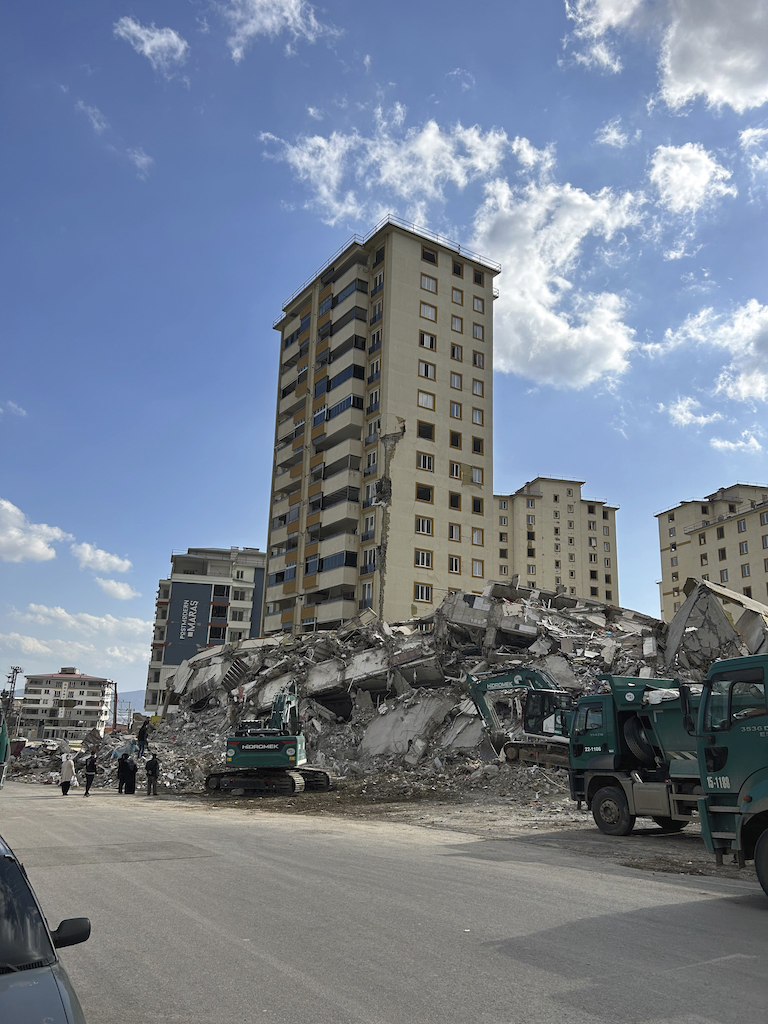
Adaptation is Necessary
In a post-earthquake environment like that following the Kahramanmaraş Earthquake Sequence, adaptation plays a necessary part in recovery. Whether that be in the form of (a) university students switching to fully remote learning, (b) hospital staff moving more critical departments to the ground floor due to a lack of elevator function, or (c) temporary installation of cell phone towers (Figure 9), finding alternative ways to get things done was a reality of the situation in the earthquake-affected region of Turkiye. That is not to distract from all the ways in which response and recovery following the Kahramanmaraş Earthquake Sequence could have been improved (of which there are many). Rather, it speaks to what the U.S. will have to expect following a major earthquake.
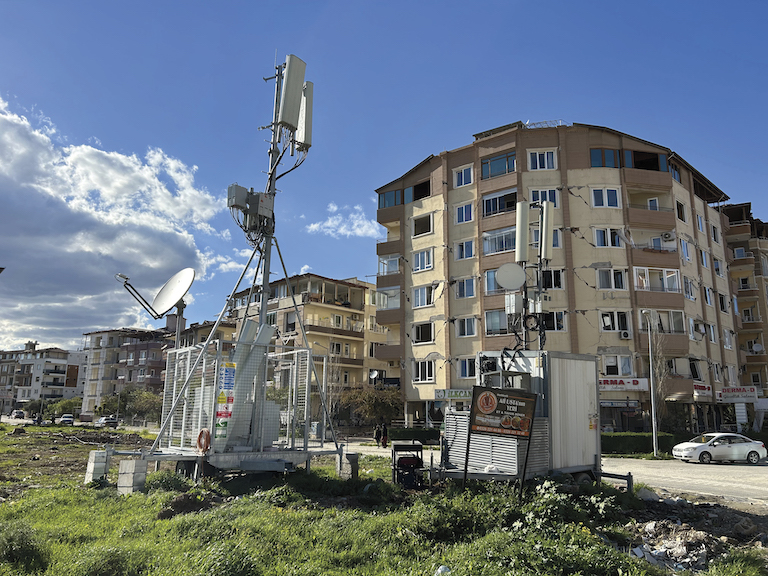
Conclusion
It is easy to fall into the mental trap of concluding that the damage and loss of life seen in the Kahramanmaraş Earthquake Sequence of February 6, 2023 are not representative of what may occur in the United States. However, the lack of a significant earthquake in the U.S. on the scale of the Kahramanmaraş Earthquake Sequence combined with much of the same underlying code philosophy between the U.S. and Turkiye may suggest otherwise. Rather than be discouraged by the observations from the Kahramanmaraş Earthquake Sequence, the authors hope that U.S. practitioners will see the opportunity to take the lessons learned from Turkiye and apply them here at home