Past, Present, and Future
The American Association of State Highway and Transportation Officials Load and Resistance Factor Design code (AASHTO LRFD) guides modern highway bridge design. The code includes prescriptive criteria for vehicular live load covering individual truck loads, lane loads, the likelihood of multiple lanes of traffic containing high truck loads simultaneously, and impact loading. Additionally, state-specific codes allow for special permit vehicles based on local conditions and needs. The current AASHTO live loads were put into practice in 1996, the latest in a series of updates developed to represent modern traffic and design practices.
Development of AASHTO Live Loads
The first written specifications for bridge design in the U.S. can be traced to railroad companies in the early 1870s. Previous to that, highway and railroad bridges were to meet simply stated criteria specific to the expected use and span length of the structure, usually by private investors teamed with engineers. For example, John Roebling’s 1846 Suspension Bridge to carry highway traffic over the Monongahela River in Pittsburgh, PA, was specified for:
Weight of the superstructure supported by cables
Four 6-horse teams, loaded with 104 bushels of coal
Weight of 100 head of cattle
As the need for highway bridges expanded, so did the need for consistent guidelines and design criteria. Individual engineers were interested in addressing the need for design standards. Theodore Cooper, previously best known as the chief inspector on the Eads Bridge over the Mississippi River, published his General Specifications for Steel Highway Bridges and Viaducts in 1884, followed by his Specification for Highway Bridges in 1890. Considered the “first authoritative specification…published and circulated,” Cooper’s publications were frequently cited as the basis of future codes and standards.
The American Railway Engineering Association (AREA, now AREMA), the American Association of State Highway Officials (AASHO, now AASHTO), and ASCE all worked on this issue – each developing separate guidelines in the early 1920s before coming together in 1924 when representatives of each, as well as the American Institute of Steel Construction (AISC), met, forming the Conference Committee and publishing the Specifications for Steel Highway Bridges in 1928.
The Specifications only addressed vehicular traffic as the primary source of transportations for urban areas. No discussion was included for trolley loads or shared structures which were common in many cities. Three traffic classes with associated “truck trains” representative of a line of trucks, and equivalent loads, were included. The “H” trucks defined here are the predecessors of the modern “HS” and “HL” loading definitions in today’s code (Table 1).
Following the Conference Specifications, the first edition AASHO Standard Specifications for Highway Bridges and Incidental Structures was published in 1931, with the same live load definitions (Figure 1). It was not until the 3rd edition, published in 1941, that significant changes were made. Bridge classification was eliminated, the “equivalent” load was changed to “lane” load, the HS truck (which represented a truck with a semitrailer) was defined, and the truck train was replaced by either a single design truck per lane or the lane loading. The 4th edition in 1944 contained additional changes, including modification of the HS truck to have variable axle spacing, modifications to lane loads to better suit continuous spans, and a change in nomenclature adding the development year to the truck designation.
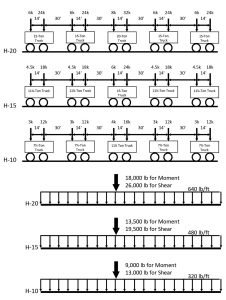
Figure 1. Specifications for Steel Highway Bridges (Conference Committee) for truck trains and equivalent loads.
Few changes were made from the mid-1940s until the development of new live load models for the AASHTO LRFD code in the 1980s. Current bridge live loads and design are based on the 1994 AASHTO LRFD code and remain basically unchanged since then.
Current AASHTO Live Load
In the 1980s and early 1990s, it became clear that the HS20 vehicle used in design was not a good representation of current highway loading and that a new design model was needed. Five candidate loads were developed and modeled using influence line analysis to look at the maximum positive bending moment, maximum shear at supports, and maximum negative moment. Representative bridges consisting of simple spans ranging in length from 10 to 200 feet and two-span continuous structures with equal spans, also ranging from 10 to 200 feet, were modeled. The goal was to determine which of the candidate vehicles would produce the most consistent results so that a single live load model could be utilized for all structure types and lengths.
The selected and current AASHTO live load vehicle is designated HL-93, and loading consists of a combination of the design truck or design tandem with the design lane load, specified to produce the extreme force effect. The total vehicle weight is 72 kips with the axle weights and spacing of the design truck as shown in Figure 2. The spacing between the two 32.0-kip axles varies between 14.0 feet and 30.0 feet.
The design tandem, representing two trailers in series attached to one truck, consists of a pair of 25.0-kip axles (50-kip total vehicle weight) spaced 4.0 feet apart, with the transverse spacing of wheels set as 6.0 feet. The design lane load consists of a load of 0.64 klf uniformly distributed in the longitudinal direction. See Figure 3 for the loading diagrams. Transversely, the design lane load is assumed to be uniformly distributed over a 10.0-foot width. The force effects from the design lane load are not subject to a dynamic load allowance.
Additionally, many states have designated Permit Vehicles required for design, which place higher loads on the structure to account for common local industry needs. The maximum legal load is the same for all states at 80 kips, while permit loads vary quite a bit with maximums up to 110 kips. Application of the permitted load varies by state with some requiring permit loads to be analyzed similarly to the HL-93, and others allowing them to be a separate load case, assuming no or limited other traffic will be on the structure at the same time.
When adapting the developed loading to long-span and multi-lane structures, it was recognized early on that the likelihood of a bridge completely packed with trucks was low, so factors were necessary to account for multi-lane traffic and the expected percentage of truck traffic on the structure. Two traffic conditions were considered in this development:
- Random traffic moving with highway speed in which the site average number of trucks is observed, evenly distributed across the structure, and are separated by an average number of cars.
- Traffic jams, with trucks moving at a slow or crawling speed in which the trucks are likely to be traveling primarily in one lane while cars utilize the others.
Truck behavior was taken from survey data from the Michigan Interstate Highways and combined with engineering judgment to develop additional influence surface models. For example, limited observation suggested that, with two lanes of traffic flowing in the same direction, about every 15th truck is on the bridge simultaneously with another truck in an adjacent lane. Based on the modeling, the multiple presence factors in Table 2 were developed and are to be applied to the live load.
These factors were based on modeling that assumed an Average Daily Truck Traffic (ADTT) of 5,000 trucks in one direction. For low traffic structures with an ADTT between 100 and 1,000, 95% of these values may be used. For extremely low truck areas with ADTT less than 100, 90% of these values may be used.
Modern live load analysis for bridge structures typically utilizes design software to determine the worst-case loading. Thousands of individual load cases can be considered on a structure to calculate the worst possible force effects on the bridge. The analysis will include load cases with the lane load covering single lane loading, multiple lane loading, single-span loading, and multi-span loading. For each condition, the truck point loads are moved throughout the loaded area to determine the location causing maximum shear and maximum positive and negative moment in the component being designed. No single load case will control the overall design of the structure.
The Future of Live Load Models
As it has now been over 25 years since the live load truck models currently used in design were developed, the question arises if these models are still applicable to modern traffic patterns and vehicle designs. To investigate this, the Federal Highway Administration (FHWA) has started to collect data on vehicle weight, frequency, and axle arrangements in various parts of the country. By looking at representative samples of Weigh in Motion (WIM) data obtained as part of the ongoing studies, one can start to get a sense of how current conditions compare to design standards.
Data obtained from interstate highway bridges around metropolitan areas in Oregon and Georgia provide a basis for quick comparisons and justification for further study. Seasonal changes in traffic are to be expected, so a year’s worth of data is required for complete analysis. However, a sample of the data looking just at April and October 2018 was analyzed using metrics of Gross Vehicle Weight (GVW) and average vehicle weight for demonstrative purposes (Table 3). Data related to axle weight, axle spacing, vehicle spacing, speed, and frequency were also collected but have not been included here.
Looking individually at the data from Georgia would imply that the current design criteria fit observed traffic reasonably well. Only 3% of the vehicles observed are over the design GVW, which could easily be explained by state permit loads. Additionally, the approximate lane load is well below the 0.640 k/ft used in design. However, looking at the Oregon data is less reassuring. With up to 9% of the observed vehicles over the design load and an additional 7% over the design tandem load, it appears that a more substantial design load may be called for. The lane load observed in this data set also approaches or exceeds the design lane load, again implying that higher design loads may be justified.
Traffic loads are likely to change significantly in the not-too-distant future as autonomous vehicles, both cars and trucks, become more common. The possibility of driverless, long truck trains, moving in close formation and high speeds, and the significantly higher load potential they present, has not yet been considered. As this becomes a reality, both codes and existing infrastructure will need to be carefully reevaluated.
Conclusion
Bridge live load modeling as prescribed in the AASHTO LRFD code has developed over the past century to account for vehicle changes, advances in modeling techniques, and new and better data on existing traffic. As traffic continues to evolve, it is both timely and appropriate that the FHWA is again looking into this matter. Preliminary data indicates that changes may be called for and validates the effort involved in the studies.■
References
Kulicki, John & Stuffle, Timothy. Final Report: Development of AASHTO Vehicular Live Loads. Submitted to the Federal Highway Administration on December 18, 2006.
Kulicki, John & Mertz, Dennis. Evolution of Vehicular Live Load Models During the Interstate Design Era and Beyond. July 11, 2006.
AASHTO LRFD Bridge Design Specification, 6th Edition. 2012.
Leech, Thomas & Kaplan, Linda. Bridges… Pittsburgh at the Point… A Journey Through History. Copyright 2016. Woodridge Publications, LLC. Word Association Publishers, Pittsburgh, PA.
WIM data provided by Intelligent Infrastructure Systems, Philadelphia, PA 2019.