As discussed previously in Untreated Submerged Timber Pile Foundations – Part 1 (STRUCTURE® magazine, December 2013), deterioration of pile tops exposed above groundwater levels is a well-known problem. It is less known that submerged portions of timber piles can also deteriorate with time, albeit at a slower rate, due to bacterial attack. This may become critical when considering underpinning methods aimed at extending service life of structures supported on timber piles. Historically, timber-pile supported structures have been underpinned by the cut-and-post method, where the top portion of the timber piles is cut and replaced with concrete posts or concrete-encased steel posts. Although the cut-and-post method appears to be relatively straight forward and simple to execute, it remains an expensive undertaking due to accessibility issues, required temporary shoring and bracing, dewatering, and labor costs. Klaassen (2008-1) reports that, in the Netherlands, foundation replacement or repair sometimes involves up to 50% of the total renovation costs for a structure. The authors’ experience in the Boston, Massachusetts area indicates cut-and-post underpinning of a typical downtown row house costs approximately $200,000 to $250,000. Bacterial attack in the remaining, submerged portion of the timber piles, however, may limit the effectiveness of the cut-and-post method, as well as the estimated remaining service life of the piles.
Remaining Service Life
The aim of any foundation remediation/repair design is similar to that of new foundation design: its design and execution must be able to (1) safely sustain all likely applied loads without failure (i.e. without overloading beyond the strength capacity of the foundation system), and (2) remain serviceable for the required use of the structure (e.g. without excessive settlement) during its intended service life. Hence, one of the greatest challenges in pile foundation remediation/repair design, and a key item for its success, is performing a reliable assessment of the current in-situ foundation’s material properties and loading history, after years in service and exposure to the surrounding natural environment. This forms the basis for the estimation of the remaining service life of the foundation system, if it is to be re-used.
The estimated remaining service life of any foundation system is governed by the determined minimum structural capacity (dependent on material properties and level of deterioration), the geotechnical capacity (dependent on soil properties and soil-structure interaction), and the magnitude of expected movements (e.g. settlement) compared to the allowable movements that a structure can sustain.
Determining Remaining Structural Capacity
Based on the review of published literature and on relevant experience, the following approach is proposed to determine the remaining structural capacity of continuously submerged timber piles:
Step 1 – Estimate the applied compressive stress acting on the timber pile cross-section versus time, considering the reduction in available load-bearing pile cross-section due to continued bacterial decay penetration. For spruce and pine piles, an estimated rate of advance of severe degradation of 0.0051 inch/year and 0.0098 inch/year, respectively, can be used (Klaassen 2009). The following considerations should be included:
- Current measured penetration of severe bacterial deterioration (based on probing to determine depth to sound wood) to be used as the starting point from which future reductions in pile cross-section will occur.
- Determine the taper of the timber pile for estimation of the pile tip diameter based on pile top diameter. Timber piles in many cases tend to derive their capacity by end bearing on a suitable soil stratum; therefore, the critical pile section is located at or near the pile tip. The rate of severe bacterial degradation should be applied uniformly over the entire pile length.
- The rate of bacterial attack decreases significantly beyond the heartwood-sapwood interface in spruce and pine piles. Therefore, for these species at least, it is reasonable to assume that for the most typical required service life of structures (i.e. 100 years or less), only the sapwood will deteriorate significantly and no further reduction in pile cross-section due to bacterial decay is expected once the sapwood thickness has been expended. The determination of the sapwood/heartwood boundary requires microscopic examination for heartwood signs, and for bacterial invasion and deterioration at different depths within the pile; this can be subjectively influenced by the examiner’s experience. Without detailed microscopic observations, the depth to the heartwood/sapwood boundary can only be roughly estimated from obvious color changes in the wood, or based on publications like The Wood Handbook (USDA, 2010) or the Textbook of Wood Technology (McGraw-Hill, 1980).
- Although the deteriorated sapwood has some measurable compressive strength, it seems prudent to ignore its contribution to the timber pile strength capacity. Measured values of elastic modulus for specimens of deteriorated sapwood obtained from piles (from previous projects) indicate that the ratio of elastic moduli between deteriorated sapwood and sound heartwood is in the range of 0.1 or less. Therefore, it would be expected that more than 90% of the applied load is resisted by the stiffer and stronger heartwood.
Step 2 – Estimate timber pile compressive strength versus time by using the reduction in compressive strength due to aging/duration of loading for heartwood shown in Figure 1. This assumes that all of the remaining pile section (based on probing to measure the depth to sound wood), including any small amount of sapwood present, can be represented by the reduced average compressive strength for heartwood.
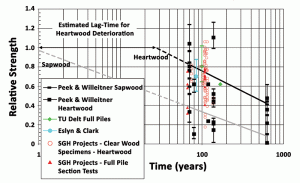
Figure 1: Decrease in timber pile compressive strength with in-service age (Base figure from Van Kuilen, 2007).
Step 3 – Determine estimated remaining structural service life of submerged timber piles by determining the time (from present) at which the demand-to-capacity (D/C) ratio for the various timber pile diameters considered (i.e. the ratio of applied compressive stress to the remaining compressive strength) is equal to the desired factor of safety level. Alternatively, the designer may choose to use a target minimum allowable percent loss in pile cross-section to determine the remaining structural service life of the submerged timber pile.
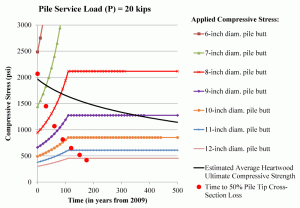
Figure 2: Sample plots of applied compressive stress and estimated heartwood compressive strength vs. time.
Figure 2 shows a sample plot of the calculated reduced heartwood compressive strength with time (Step 2), superimposed on plots of the applied compressive stress curves developed for various timber pile diameters as bacterial decay penetrates to the heartwood/sapwood boundary for a service load of 20 kips (Step 1). The intersection of the time under load dependent ultimate heartwood compressive strength curve and the applied compressive stress curves defines the expected remaining pile service life for each pile diameter at the expected load combination, with no factor of safety included. Figure 2 was developed for spruce piles with an in-service age of 109 years, 2-inch sapwood thickness, and measured severe bacterial attack penetration of about 0.75 inches at time zero. The plot of applied compressive stress is shown in relation to the pile butt (pile top) diameter, rather than the critical pile section (i.e. pile tip) used to calculate the applied stresses for ease of use during investigations where, typically, just the pile tops are exposed. Figure 2 also shows the time for 50% loss of original pile cross-section for reference.
Figure 3 shows plots of the estimated remaining service life for each timber pile butt (top) diameter and different levels of applied service load calculated similarly to Figure 2, and considering a D/C ratio of 1.0 (i.e. no factor of safety is included). Since Figure 2 only considers time up to 500 years, the curves in Figure 3 level off at 500 years.
Evaluation of Remaining Geotechnical Capacity
The Table summarizes the results of load testing on five timber piles from separate areas of a single project site in downtown Boston. Two of these piles were extracted after the load tests were performed.
Results of the pile load tests indicate no apparent adverse impact of timber pile deterioration on the geotechnical bearing capacity of the piles. Area 1-1, which showed a larger penetration depth of severe deterioration (with 75% or more loss of pile cross-section), performed stiffer and had a higher measured unit end bearing capacity than the Area 1-2 pile which showed less deterioration (about 34% loss of pile cross-section). However, the data evaluated in this analysis is too limited to draw more in-depth conclusions regarding the impact of deterioration on the geotechnical capacity of the submerged timber piles.
Estimated Future Settlements
Once cut-and-post underpinning is performed (i.e. no inelastic settlement due to softening of pile tops), and assuming no change in the level of applied loads or in soil or groundwater properties, the only viable mechanism for future settlement is elastic compression of the remaining submerged timber pile sections. This can be due to potential softening as a result of aging/creep, and decay (i.e. a decrease in the Young’s modulus, E) of the timber piles. The National Design Specification® (NDS®) for Wood Construction (2005) recommends a creep factor of 2 for wet wood, i.e. the E value should be decreased by 50% under long-term permanent loads. Current design standards do not provide recommendations for further reductions in E values due to decay.
A review of limited data available from compressive-strength testing of timber pile samples from various projects throughout northern U.S. (with in-service ages ranging from about 100 years to 137 years) indicates that there may be an ongoing reduction in E values with time, similar to that of compressive strength values. However, the data spread is too broad and the breadth of time periods too limited to be able to more accurately and reliably infer a rate of degradation of the E value with time.
Assuming a 50% loss of cross-section in the timber piles, a pile length of about 10.5 feet, and using an E value of 1.2 x 106 psi for spruce (average published E value for fresh spruce from ASTM D2555-06), the added submerged pile settlement under sustained loads varies from less than 0.04 inches (for a service load of 50 kips combined with a 12-inch pile diameter), to about 0.2 inches (for a service load of 50 kips and a 6-inch pile diameter). Even if it is assumed that, over time, the E value has degraded to about 50% of its original value throughout the pile length, the added submerged pile settlement under sustained loads for the same assumed conditions remains low, varying from about 0.1 inches to 0.4 inches. If the applied loads are sufficiently high, longer pile lengths could result in settlements greater than 0.5 inches.
Based on the calculation case described above, added settlement solely due to pile elastic compression will likely not exceed 0.5 inches over the remaining service life of timber piles. However, detailed settlement calculations, taking into account actual pile diameters, pile lengths, measured pile properties and applied load magnitudes, must always be performed. Although most structures can experience differential settlements on the order of 0.5 inches without resulting in much structural distress, the foundation remediation designer will have to take into consideration the present condition of the structure. If the structure is fragile and has already undergone significant settlements, even small added settlements could have an adverse impact on its serviceability.
Conclusions
- The remaining service life of in-service timber piles appears to be controlled by the structural capacity of the timber piles, rather than their geotechnical capacity. Evaluation of a more significant amount of data is necessary for confirmation of this postulate.
- Measured rates of bacterial deterioration indicate that, for piles with 100 to 140 years of in-service age and with diameters of 6 inches or less, bacterial decay may have advanced sufficiently that little to no remaining service life is anticipated. For relatively small applied service loads (around 10 kips per pile and no factor of safety included), pile diameters of 7 inches or greater are likely have a remaining service life of 100 years or greater from present time. For larger applied loads (on the order of 40 to 50 kips per pile), pile diameters of 10 inches or greater would be required to attain the same remaining service life expectancy (100 years or more).
- Once tops of piles are replaced and the new pile cutoffs remain submerged (e.g. cut-and-post underpinning is performed), settlements due to pile elastic compression over the remaining service life of the timber piles will likely not exceed 0.5 inches.
The analysis used herein to estimate remaining service life of submerged timber piles is based on average conditions (i.e. average measured strength and/or modulus values). Although measured strength and modulus data is well distributed around the average values used, there is still a 50% probability that the actual values may be lower or higher than the ones used. In addition, other than limiting the depth of penetration of bacterial decay to include only the thickness of the sapwood of submerged piles, it is possible that local building codes may require foundation remediation/repairs be performed once a certain percentage of the original pile capacity has been lost. For the smaller-diameter timber piles, this would likely result in smaller remaining service life expectancies than those indicated above.
Final Thoughts
Further research remains to be performed regarding the impact of bacterial attack on submerged timber pile structures, especially any potential reduction of the pile’s geotechnical capacity.
There is also continued concern that soft-rot deterioration could still occur even with groundwater levels maintained above the top of the untreated pile cutoff. Recent research indicates that soft-rot attack may be supported even in submerged conditions, if the dissolved oxygen content in the groundwater is above a threshold value of 2 ppm (Klaassen 2005). Given that potable water is often used for recharging groundwater levels near timber piles to maintain submersion, and this could lead to an increase in dissolved oxygen levels in the groundwater, further research is required to confirm this potential deterioration mechanism.
Development of a large database of U.S. historic building stocks supported on untreated timber piles, similar to that currently in existence for some European countries, would be of significant value in evaluating current conditions and required foundation remediation/repair options. Based on the European studies on bacterial decay, existing untreated submerged small-diameter timber piles with more than about 100 years in service (which represents a significant percentage of the existing untreated timber pile stock in the U.S.) are likely to be reaching a level of bacterial attack at which there is little to no remaining service life. For these structures, significant structural settlement, with the consequent building distress, may start developing within a relatively short-time from present.▪
References
Boutelje, J.B., and Bravery, A.F. (1968), Observations on the Bacterial Attack of Piles Supporting a Stockolm Building, J. Inst. Wood Science, 4(2):47-57.
Huisman, D.J., Kretschmar, E.I., and Lamersdorf, N. (2008), Characterising Physicochemical Sediment Conditions in Selected Bacterial Decay Wooden Pile Foundation Sites in the Netherlands, Germany and Italy, International Biodeterioration and Biodegradation Vol. 61:117-125.
Klaassen, R.K.W.M (2008-1), Bacterial decay in wooden foundation piles – Patterns and causes: A study of historical pile foundations in the Netherlands, International Biodeterioration and Biodegradation Vol. 61:45-60.
Klaassen R.K.W.M. (2008-2), Water Flow Through Wooden Foundation Piles: A Preliminary Study, International Biodeterioration and Biodegradation Vol. 61:61-68.
Klaassen R.K.W.M. (2009), Factors that influence the speed of bacterial wood degradation, International Conference on Wooden Cultural Heritage, Hamburg, October 2009.
Hoyle and Woeste (1989), Wood Technology in the Design of Structures, 5th Edition, Iowa State University Press.
Meyerhof, G.G. (1976), Bearing Capacity and Settlement of Pile Foundations, J. of the Geotechnical Engineering Division, GT3, March 1976.
Nilson, T. and Bjordal, C. (2008), Culturing Wood-Degrading Erosion Bacteria, International Biodeterioration and Biodegradation Vol. 61:3-10.
ASTM D245 – 06, Standard Practice for Establishing Structural Grades and Related Allowable Properties for Visually Graded Lumber.
ASTM D2555 – 06, Standard Practice for Establishing Clear Wood Strength Values.
ASTM D2899 – 03, Standard Practice for Establishing Allowable Stresses for Round Timber Piles.
National Design Specification (NDS) for Wood Construction with Commentary and Supplement: Design Values for Wood Construction 2005 Edition, ANSI/AF&PA NDS -2005, American Forest & Paper Association, Inc.
Vatovec, M., and P.L. Kelley, Biodegradation of Untreated Wood Foundation Piles in Existing Buildings – Remedial Options, STRUCTURE magazine, Dec. 2007.
Vatovec, M., and P.L. Kelley, Biodegradation of Untreated Wood Foundation Piles in Existing Buildings – Deterioration Mechanisms, STRUTURE magazine, Sept. 2007.
Vatovec, M., and P.L. Kelley, Biodegradation of Untreated Wood Foundation Piles in Existing Buildings – Investigation, STRUCTURE magazine, June 2007.
Wood Handbook – Wood as an Engineering Material. U.S. Department of Agriculture, Forest Service, Forest Products Laboratory. General Technical Report FPL-GTR-190. Madison, WI: U.S. 2010.
Textbook of Wood Technology – Structure, Identification, Properties, and Uses of the Commercial Woods of the United States and Canada, Fourth Edition. Panshin, A.J. and de Zeeuw, C., McGraw-Hill, 1980.